1. Introduction
In Uruguay, pears and apples are harvested from January or February to April, respectively. In order to provide fruit throughout the year, and maintain quality and health, apples and pears are stored in cold chambers at 0-1 ºC. Even in these conditions, fungal rots are developed. The main pathogen associated to fungal rot in apples and pears stored at cold temperature is Penicillium expansum, which is the main causal agent of blue mold1. However, other fungi such as some species of Alternaria (A. alternata, A. tenuissima and A. arborescens) or Botrytis cinerea are also involved in fungal postharvest diseases in both types of fruit2)(3)(4.
In general, the prevention of postharvest diseases is achieved using fungicides, which are applied to the fruit before they are stored. However, the reduction of maximum residue limits in food and the concern about the environmental fate of pesticides has encouraged the search for alternatives to control fungal diseases. In this regard, biological control appears as a feasible alternative. In particular, yeasts have shown a great potential as biocontrol agents of postharvest diseases5. A great number of yeasts strains, belonging to several species, have been tested as biological control agents, achieving in some cases a commercial formulation6. Despite the development of some commercial products, the search for novel biological control agents of postharvest diseases keeps going.
Particularly, to prevent the common fungal postharvest diseases in apples and pears during cold storage, some characteristics should be considered in the primary selection of a biocontrol agent.
Since antagonists are intended to protect fruit wounds from fungal attack during the storage at low temperatures, the selection should be oriented to cold adapted microorganisms with the ability to colonize fruit wounds, which are the main infection court of necrotrophic, decay pathogens. Moreover, the inability to produce pectinolytic enzymes that could degrade fruit tissue should be also considered in the selection7. Various mechanisms, including nutrient and space competition, the production of soluble or volatile inhibitory metabolites or enzymes to degrade the pathogen cell walls (involved in hyperparasitism), and the induction of host resistance have been postulated to play a meaningful role in the biocontrol of postharvest pathogens by microbial antagonists8)(9)(10)(11)(12)(13)(14)(15)(16. Screening of new antagonists has been extensively based on in vitro tests leading to detect the production of antimicrobial compounds, metabolites or enzymes related to the mechanisms of action mentioned above. These tests are fast and easily performed and have led to a bias in the selection of biocontrol agents17)(18.
In previous studies, our group isolated, identified and characterized cold tolerant yeasts from soil and water from King George Island, Antarctica19. A collection of 61 psychrotrophic yeast strains, characterized by their ability to produce various extracellular enzymes (including amylases and pectinases), was obtained. Debaryomyces hansenii was the most abundant species. Among other common characteristics, all the strains of this species share the particularity of being unable to produce pectinases or amylases. D. hansenii is characterized at species level by its tolerance to extreme conditions, such as low pH, high osmotic pressure, oxidative stress and low temperature20. These characteristics make D. hansenii a good candidate to survive and proliferate in fruit wounds under packinghouse conditions where a variety of abiotic stresses, such as oxidative stress, lack of nutrients, low temperature and adverse pH, could limit microbial growth21.
Many strains of D. hansenii have been selected as biological control agents of fungal diseases on various fruits during postharvest storage. Successful control of P. digitatum in grapefruit22, Geotrichum citri-aurantii in Mexican lime23, P. italicum24 and P. digitatum in lemon fruit25, oranges and mandarins26, Colletotrichum gloeosporioides in papaya27, and Monilinia fruticola in apples20 has been achieved by the use of D. hansenii strains as biocontrol agents. In most cases, protection has been assessed at room temperature; however, in the case of apples and pears, a good biocontrol activity at 0-1 °C is needed to prevent rots after a long period of cold storage. In this work, 16 D. hansenii isolates from Antarctica were evaluated as biocontrol agents of P. expansum in apples and pears during cold storage. Only one strain was selected due to its ability to reduce blue mold incidence in both apple and pears. Several mechanisms of action, such as the production of chitinases, siderophores and soluble and volatile antifungal compounds, the ability to form biofilms and the capacity to inhibit the germination of P. expansum spores, were evaluated in order to elucidate if any of these characteristics could be related to biocontrol activity on fruit. To allow the differentiation of the selected strain from others from the same species a specific molecular profile was obtained by ISSR-PCR.
2. Materials and methods
2.1 Biocontrol agents and pathogen
The 16 Debaryomyces hansenii strains used in this study belong to a collection of yeasts isolated from soil and water samples from King George Island, South Shetland Islands, Antarctica19. Cultures are maintained in Eppendorf tubes containing Potato Dextrose Agar (PDA) stored at 4 °C. These strains are non-pectinase-producing strains. A native strain of Penicillium expansum (P17) previously isolated from rotten apples and identified at specie level28 was used in the current study.
2.2 Fruits
Red Delicious apples and Williams pears used in this study were obtained from a local orchard (Granja Moizo, Montevideo, Uruguay) within a month after harvest. Fruits were selected by uniformity, size, color, shape and visual absence of damage or fungal growth.
2.3 Fruit juice growth ability at 0 ºC
The ability of D. hansenii strains to grow in apple juice and pear juice at low temperature 0-1 °C was evaluated. A 250 mL flask containing 50 mL of steam sterilized apple or pear juice was inoculated with a suspension of each yeast to reach a final concentration of 1(104cell mL-1 and incubated at 0-1 °C for 30 days. Microbial growth was evaluated by cell count in a Neubauer chamber. Each assay was repeated three times.
2.4 Biocontrol assay
The yeasts were evaluated for their biocontrol ability against Penicillium expansum in wounded Red Delicious and Williams pears stored at 0-1 °C. Fruits were first surface-disinfected with 70% ethanol. After drying, five wounds (4 mm deep ( 2 mm wide) were made along the equator of each fruit. Each wound was then inoculated with 10 μL of a yeast saline suspension (1(107cell mL-1) made from a two-day-old PDA culture. Control wounds were inoculated with 10 μL of sterile saline. Wounds were allowed to dry at room temperature and then subsequently inoculated with 10 μL of a 1(104conidia mL-1 conidial suspension of P. expansum in saline solution with Tween(80 (0,1 %). Six fruits (5 wounds per fruit) were used per treatment (n = 30 wounds). Fruits were stored in a cold chamber at 0-1 °C until control wounds showed rot development. Disease incidence (measured as the percentage of infected wounds) and disease severity (measured as the percentage of treated wound diameter compared with control wound diameter) were evaluated.
2.5 Yeast characterization
2.5.1 Yeast growth in fruit wounds
Fruits were surface disinfected with ethanol 70% and let dry in a laminar flow hood. Three wounds (2mm ( 2mm ( 4mm) per fruit were made, and each wound was inoculated with 10 µL of yeast suspension (1(107cell mL-1). After let dry at room temperature, fruits were incubated in a cold chamber at 0-1 °C during 60 days in the case of apples and 45 days for pears. After incubation time, wounds were removed using a sterile scalpel and placed in eppendorf tubes containing 1 mL of Tween 80 solution (0,1% w/w). Tubes were mixed in a vortex for 5 minutes and 10-fold serial dilutions were made. Serial dilutions were poured in PDA plates (Potato Dextrose Agar). Plates were incubated at 25 °C during 48 h and colonies were counted. Each assay was repeated three times.
2.5.2. Chitinase production
Chitinase production was evaluated in YNB (Yeast Nitrogen Broth) containing 0.5% colloidal chitin and 2% agar. Colloidal chitin was prepared using ground chitin (Sigma-Aldrich)29. Petri dishes were inoculated with a strip of each yeast and incubated at 25 °C for 48 h. Chitinases production was visualized as a halo surrounding the yeast strip and recorded as positive or negative producer.
2.5.3. Siderophore production
Siderophore production was evaluated in CAS (Chrome Azurol Sulfonate) agar plates30. Each isolate was streaked in a section of the plate and incubated at 25 °C for 48 h. After incubation, siderophores production was evidenced by a color change from blue to orange surrounding the streaks of growing cultures and recorded as producer or not producer.
2.5.4. Production of soluble and volatile antifungal compounds
The ability of each strain to inhibit P. expansum growth was tested in dual cultures. The soluble antifungal compounds production was evaluated in Petri dishes (9 cm diameter) filled with apple juice agar (AJA, apple juice + 2% agar)31. Yeast was streaked at 2 cm of the rim of the plate and a non-sporulated agar plug of P. expansum (8 mm diam.) from a 2-days-old PDA culture was inoculated on the same plate at a distance of 5 cm from the yeast line. The pathogen colony diameter was measured after incubation. Three replicates for each treatment were used.
The production of volatile antifungal compounds was tested as described in a previous work7. Plates containing AJA were inoculated centrally with a non-sporulated agar plug of P. expansum. At the same time, another plate containing AJA was superficially inoculated with a yeast suspension, which was evenly spread on the surface with a sterilized glass spreader. The lids of plates were removed, and the double dish set was sealed using Parafilm. Control plates were inoculated with pathogens but covered with AJA plates not inoculated with yeast. The closed plates were incubated at 25 °C for 3 days. The pathogen colony diameter was measured after incubation. Three replicates for each treatment were used.
2.5.5. Biofilm formation
The assay was carried out in a 96-well microtiter plates (Montegrotto Terme, Padova, Italy); each plate was filled with 180 μL of sterile apple juice and 20 μL (1(107cell mL-1) of a yeast suspension, prepared in sterile water from a 2-day-old PDA culture. The plate was incubated for 3 days at 25 ºC. The control consisted of wells containing only sterile apple juice. After incubation, the wells were emptied, rinsed with water, and air-dried at room temperature. The adherent biofilm layer was stained with an aqueous solution of violet crystal 1% (w/v) for 20 min, rinsed with water, and air-dried32. The bound dye was eluted from each well with 200 (L of an ethanol:acetone solution 80:2033. The absorbance of each well was measured at 590 nm. Each treatment was repeated three times in the same plate.
2.5.6. Inhibition of spore germination by D. hansenii strains
Penicillium expansum spore germination curve at low temperature was performed in a 96-well microtiter plate. Each well was filled with 100 μL of sterile apple juice and 10 μL of spore suspension (1(106 spores mL-1, prepared from a 1-day-old PDA culture). The plates were incubated at 0-1 °C and spore germination was evaluated daily by observing microscopically 100 spores. A spore was considered germinated when the germ tube was greater than the spore diameter. Each experiment consisted of three replicates. The effect of D. hansenii yeasts on spore germination of P. expansum was carried out in a 96-well microtiter plates. Each plate was filled with 100 μL of sterile apple juice, 10 μL of a yeast suspension (1(107cell mL-1, prepared in sterile water from a 2-day-old PDA) and 10 μL of a spore suspension (1(106spores mL-1, prepared from a 1-day-old PDA culture). The plate was incubated for 10 days at 0-1 °C and the spore germination was compared with the control (wells without inoculation of yeasts) as detailed before.
2.6 ISSR-PCR
DNA extraction method was performed as described by Schena and others34. Yeast ISSR-PCR profiles were obtained by PCR amplifications performed in a 25 μL reaction volume containing 1 μL of DNA, 1 μL of primer (25 μM), 2.5 μL of dNTP (Bioron, 2.5 mM), 2.5 μL of Taq Buffer (Thermo Fisher Scientific Inc., USA), 0.1 μL of DreamTaq DNA polymerase (Thermo Fisher Scientific Inc., USA) and 17.9 μL of miliQ water. The tested primers are shown in Table 1. All amplifications were carried out using a MultiGeneTM thermocycler (Labnet International Inc., Edison, NJ, USA). The cycling conditions were as follows: initial denaturation step at 94 °C for 1 min, 40 cycles of denaturation at 94 °C for 1 min, primer annealing (at 45 °C for primer (GACAG)3 and 50 °C for primers (AGG)5 and (CAG)5) for 2 min, and extension at 72 °C for 2 min, followed by a final extension at 72 °C for 5 min. Electrophoresis was performed with 5 μL of amplified products on a 1.5% agarose gel at 80 V.
2.7 Statistical Analyses
Analysis of variance (ANOVA) of data was performed using InfoStat software package35, and Tukey test was used to determine differences between means at 0.05 significance level. Statistical analysis of disease incidence was performed using a generalized linear model, assuming a binomial distribution, with a logit transformation (maximum likelihood). Treatments were compared by the DGC test36 at a significance level of 0.05 using the InfoStat software package35.
The correlation between inhibition of spore germination and blue mold incidence reduction on fruit was determined by means of Spearman correlation coefficient, using Infostat software package35.
3. Results and discussion
3.1 Fruit juice growth ability at low temperatures
The 16 yeasts were able to grow in apple and pear juice at 0-1 °C. The ability to grow at low temperatures was expected and it was the reason why we decided to isolate yeasts from a cold environment like Antarctica. Growth rate and cell concentration in the stationary phase are shown in Table S1 (Supplementary material). The lag phase was between 3 and 5 days in apple juice, and between 4 and 6 days in pear juice. The stationary phase was achieved between 15 and 23 days after inoculation.
For most biocontrol agents, except for those that act by inducing resistance, the ability to grow in the site where the interaction with the pathogen is going to take place is a particularly important trait37. In the case of yeasts intended to protect apple and pear wounds from fungal attack during postharvest storage in cold chambers, the ability to grow in fruit juice at low temperature 0-1 °C is an experimental approach to determine if they are adapted to grow in such stressful conditions (low pH, high sugar concentration and low temperature). In this case all the strains could grow at the practically same rate, so they were all considered for further analysis.
3.2 Biocontrol assays
Biocontrol assays on fruit revealed phenotypic differences among D. hansenii strains. This result is in accordance with previous works7)(38 where it was demonstrated that biocontrol activity is strain specific and not an ability necessarily shared by strains from the same species. In Red Delicious apples, D. hansenii strains were able to significantly reduce (p = 0.05) the disease incidence and severity relative to the control (wounds inoculated only with the pathogen) after 60 days of incubation (Fig. 1). However, only 10 strains reduced blue mold incidence in more than 75%. In case of pears cv Williams, P. expansum rot was significantly reduced (p = 0.05) by 13 strains, and only one strain (F9D) could achieve control levels over 75% (Fig. 2A). Despite reduction of disease incidence achieved by yeasts in pears was low, in most cases severity in treated fruit was significantly lower than the observed in the control (Fig. 2B).
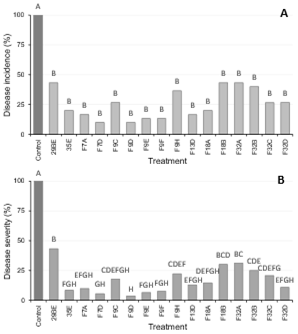
Figure 1: Average percentage disease incidence (A) and severity (B) in Red Delicious apples inoculated with a yeast suspension, followed by inoculation of a Penicillium expansum conidial suspension. The control was not treated with a yeast. Treatments with the same letter are not significantly different (p=0.05), n =30 wounds
Biocontrol activity of most yeast isolates was dependent on the host fruit. A good biocontrol activity against P. expansum in apple was not related to the same performance on pears. These differences could be attributed, among others, to different availability of nutrients and pH in fruit, different P. expansum susceptibility or physiological changes during fruit storage. Our results are in accordance with previous works in which it was demonstrated that the host had a high influence on the biocontrol activity of microbial antagonists39)(40.
In the present work, strain F9D was selected since it had a good performance controlling blue mold rot on both apples and pears. The ability to prevent fungal rot in various commodities is a desirable trait of a biocontrol agent. It would allow a single product based on its activity to have a wider range of applications and to increase its market potential32.
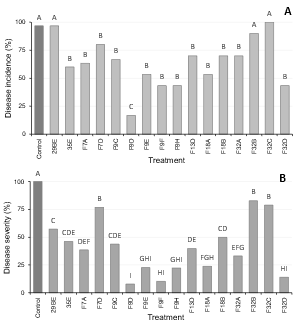
Figure 2: Average percentage disease incidence (A) and severity (B) in Williams pears inoculated yeast suspension, followed by inoculation of a Penicillium expansum conidial suspension. The control was not treated with a yeast. Treatments with the same letter are not significantly different (p=0.05), n=30 wounds
3.3 Yeast characterization
To understand differences among D. hansenii strains with different biocontrol activity, characteristics usually associated with biocontrol potential were determined. None of the assayed yeasts produced soluble antifungal compounds active against P. expansum in apple juice agar. In all cases, in dual cultures, P. expansum growth covered all Petri dish in 3 days, as in the control plate.
Production of volatile antifungal compounds was neither detected. Neither of the strains produced siderophores nor chitinases in the assayed conditions, while five strains (35E, F7D, F18B, F32A, F32C) exhibited biofilm production when grown in apple juice. Many mechanisms of action, including the production of antifungal organic compounds (volatile or soluble)27)(41, biofilms, siderophores and chitinases42, have been reported to explain the biocontrol ability of different Debaryomyces hansenii strains. However, Debaryomyces hansenii has been categorized by Breuer and Harms43 as a highly heterogeneous species due to the phenotypic differences between strains, such as variations in optimal growth conditions, versatile assimilation patterns of carbon sources and enzymes production.
Moreover, it has been demonstrated that in the case of D. hansenii, production of chitinases, siderophores and biofilm formation is a strain specific trait42. D. hansenii has been frequently reported to produce killer toxins, proteins with antimicrobial activity which can be detected in dual culture assays. D. hansenii killer toxins have shown antimicrobial efficiency towards several yeast species, in particular C. albicans44, some Gram positive and Gram negative bacteria and some pathogenic fungi such as A. alternata, M. fructicola and M. fructigena45)(46. Although killer toxins in Debaryomyces hansenii are probably encoded by chromosomal genes47, it has been demonstrated that their production is strain specific48. Moreover, killer activity is highly dependent on the yeast growth conditions (pH, NaCl concentration and temperature) so it could have not been expressed in apple juice and probably would not be expressed in apple wounds at 0-1 °C.
The production of volatile antifungal compounds by strains of D. hansenii has also been reported elsewhere27)(46. Yeasts can produce many volatile metabolites, as result of their primary and secondary metabolism. In general, VOCs obtained from primary metabolism are produced as a means of detoxification, while those produced by secondary metabolism pathways are involved in defense or communication mechanisms49. In both cases, VOCs production depends on the strain7 and on the growth conditions.
Our results demonstrated that biocontrol activity of D. hansenii strains is not based on the production of antifungal metabolites. This could be considered a good result in the selection of a biocontrol agent. If such metabolites are involved, potential toxicological risks should be assessed. Moreover, antagonism based on antimicrobial activity could be not durable due to the appearance of less sensitive pathogen strains17.
Pathogen conidia germination is a preliminary step of host colonization. This event largely depends on temperature, humidity of the environment and presence of compounds released by the host50. The inhibition of germination of P. expansum spores was evaluated for each strain in fruit juice at low temperature 0-1 °C. A certain degree of inhibition was detected in all cases (4 to 54%) (Fig. 3). However, a significant correlation between the biocontrol activity of the strains in apple and pear and their ability to inhibit the germination of P. expansum spores could not be established by Spearman's correlation analysis (p=0.0900; p=0.1480).
Many authors have reported that competition for space and nutrients is the main mechanism by which yeasts repress the development of pathogens. In our study, yeasts growth in fruit wounds stored at 0-1 °C was evaluated after 60 days in the case of apples and 45 days in the case of pears (Table S2, Supplementary material). All yeasts were able to grow in fruit wounds reaching populations between 0,4(106 and 5,2(106 cells per wound. Similar but significantly different colonization abilities were observed among the strains. In all cases the ability to grow in apple wounds was the same or greater than that in pears (Table S2).
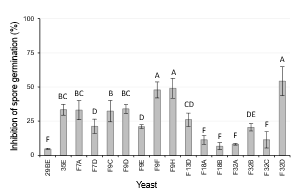
Figure 3: Inhibition of P. expansum spores germination (%) in presence of D. hansenii strains. Evaluation after 10 days of incubation at 0-1 °C. Treatments with the same letter are not significantly different (p=0.05). Error bars represent sample confidence interval (p=0.05)
Strain F9D, which was selected as the most effective antagonist in apples and pears, was not the strain with the highest colonization ability. These results led to the conclusion that other mechanisms should be involved in its biocontrol potential.
The same result was reported when studying the biocontrol potential of different Aureobasidium pullulans strains29. In that case, the authors hypothesized that the ability to produce chitinase could be related with a higher biocontrol activity. In case of strain F9D, any of the mechanisms studied in this work seems to be the basis of its biocontrol activity in apple and pear wounds. F9D did not exhibit any of the properties related to those mechanisms of action, except for the inhibition of spore germination, which was 34.2 ± 4.3%. Further studies are needed to try to explain its outstanding performance in protecting fruit wounds against blue mold rot during cold storage. Its activity could be based on a combination of various mechanisms which are not easily expressed in nutrient media, such as induction of host resistance. This mechanism should be considered, since yeast antagonists have been reported as elicitors in the interactions with fruit hosts51. However, such studies are complex, because induction of resistance depends on a sequence of events such as the production of microbial signaling compounds leading to metabolic events in the host, resulting in the induction or priming of different defense mechanisms17. The knowledge of the exact mode of action of biocontrol agents in a determined pathosystem would help to improve their action and to direct the search for new antagonists. However, most studies have concluded that biocontrol is the result of multiple mechanisms involving interactions of antagonists, pathogens, hosts, environment conditions and epiphytic microbiota. According to this, screening of new biocontrol agents should be based on bioassays on plants or plant tissues, in the same conditions in which biocontrol is expected to occur. Nevertheless, until now, many studies base the selection of antagonists on the verification of some of the mechanisms of action mentioned above, especially on the ability to produce soluble antifungal substances on nutrient media in laboratory conditions.
Our work has confirmed that the selection of a biocontrol agent should not be based on a specific mechanism of action. If our selection of antagonists had been based on their ability to produce antifungal compounds on culture media, the strain F9D would not have been found. Thus, screening processes to find biocontrol agents should be based on suitable bioassays in the corresponding pathosystem under the environmental conditions where the interaction will occur. Alternative assays based on specific mechanisms of action based on in vitro tests should be avoided. An inappropriate screening process can lead to a laborious, expensive, and ineffective selection32.
3.4 ISSR-PCR
To monitor the fate of a biocontrol agent after application, a strain specific molecular marker is a fundamental tool. It could also facilitate the registration process providing a profile which allows to differentiate the specific strain from others of the same species. In this work ISSR-PCR was evaluated as a method to type D. hansenii F9D, which resulted the selected biocontrol agent.
Three different primers were tested to determine differences among D. hansenii strains. An important genetic heterogeneity within the species was reported previously52)(53. However, the discriminative power of the ISSR-PCR method used in this work for strain typing was not high. Only when the primer (GACAG)3 was used a fingerprinting pattern specific for strain F9D could be obtained (Fig. 4).
4. Conclusions
Among 16 cold tolerant D. hansenii strains able to grow at low pH and high sugar concentration in apple and pear wounds, only one was selected for its ability to prevent blue mold on fruit during cold storage. The mechanisms by which the selected strain exerted P. expansum biocontrol on fruit could not be revealed by in vitro tests. Further studies are required. Screening methods to find new biocontrol agents to protect fruit wounds during postharvest storage should not be based on in vitro tests. Otherwise, good biocontrol agents such as D. hansenii F9D could be discarded.
Although deeper studies should be performed, this strain could be a good candidate for the development of a yeast-based formulation to protect both types of fruit.