1. Introduction
Barley (Hordeum vulgare L.) is one of the most important cereal crops in the world. It is mainly used for feed, food, malting, and brewing1. In Uruguay, it represents the second most cultivated cereal and is mostly exported as malt2. Ramularia leaf spot (RLS), characterized by reddish brown, rectangular lesions on both sides of the leaf, often surrounded by a chlorotic halo3, is caused by Ramularia collo-cygni (Rcc4). RLS is a major constraint to production in most barley growing regions, especially in the southern cone of South America5. RLS causes significant losses in both grain yield and quality 3)(5) with yield reductions up to 70%6.
In-field diagnosis in early crop stages is problematic because RLS has an asymptomatic phase. RLS lesions on leaves may be visible earlier in the growing season if environmental conditions have been conducted 3)(5) 6. Factors such as alternating wet and dry days, high light intensity, waterlogging, drought, nutrient deficiencies, and prolonged leaf-wetness contribute to varying incidence and severity of RLS 7)(8) . However, typical symptoms are commonly visualized under field conditions after the awns peeping stage (GS47-49)3)(9
Rcc is a fungus with the ability to feed on and remain associated with barley stubble 10)(11) . The epidemiological significance of stubble in relation to this disease remains uncertain. While barley seeds represent a primary source of inoculum for RLS12, quantifying seed-borne inoculum in commercial seed batches faces limitations due to reliance on qPCR techniques 13)(14) . So far, most barley varieties cultivated globally are susceptible or moderately susceptible to RLS5. Despite efforts, no commercially available fungicide seed treatment has proven efficacy against RLS5. While various elicitor combinations have been explored for their impact on RLS, their effectiveness remains limited15.
As a result, fungicides are currently the only corrective management available to control RLS in barley crops 5)(16) . Effective control has been achieved using three single-site fungicide classes, including succinate dehydrogenase inhibitors (SDHIs), demethylation inhibitors (DMIs), the quinone outside inhibitors (QoIs), as well as multi-site inhibitors, such as chlorothalonil or folpet17. However, the problem of RLS management has been exacerbated by shifts in Rcc populations' sensitivity to these fungicide groups in Europe 18)(19) 20. The European Food Safety Authority decision not to renew the approval for chlorothalonil due to environmental and health risks has further complicated the situation21.
Meanwhile, in South America, resistance to QoI was reported in Argentinean populations of Rcc. In addition, some isolates of Rcc showed a sensitivity reduction in mixtures including SDHI or DMI16. Under Uruguayan field conditions, lower or no efficacy of QoI on Rcc has been documented6. Mixtures containing SDHI, DMI and/or chlorothalonil are recommended at disease onset when environmental conditions are conducive to RLS10. Continued favorable conditions post Rcc detection at GS30-33 might need a second application during the awns peeping stage (GS47-49) 6)(10) 16. However, under less conducive conditions, single applications in the period from elongation (GS31) to booting (GS49) have shown acceptable efficiency control 6)(10) .
Chemical control strategies can result in pesticide residues on and in plants, even when applied following Good Agricultural Practices (GAPs)22. Experiments on residue behavior are necessary to determine their levels and to assess their impact on human and animal health23. To assure food and feed safety, international organizations such as the European Union24 and the Codex Alimentarius25 have established Maximum Residue Limits (MRLs) for pesticides in cereal grains, which are regularly evaluated and adjusted. Many studies have focused on the development and validation of methods for the determination of pesticide residue in grains, on residue dissipation, and also on the dietary risk assessment of these compounds in grains 26)(27) . However, only few works have tested the compliance between pesticides use and MRLs in crops under commercial field conditions 28)(29) 30 or have assessed the impact of different management alternatives. Thus, striking a balance between effective pest management and ensuring food safety remains a critical challenge.
This study aimed to evaluate the efficacy of recommended fungicide management practices used for RLS control and their fungicide residues in barley grain. There is an important challenge to jointly evaluate and discuss the effectiveness of fungicide management for RLS control and the levels of residues associated with these strategies.
Nowadays, very limited information is available on fungicide residues in cereals grain and food safety, and this is rarely taken into account by farmers when deciding on chemical control programs. In addition, international regulations are becoming more restrictive, so this information would contribute to the management of RLS considering the upcoming commercial scenarios.
2. Materials and methods
2.1 Field experiments
Five field experiments were carried out from 2016 to 2018 under different environments in barley production regions of Uruguay. The experimental sites were selected to be representative of barley production, considering factors such as climatic differences among areas.
Two trials were located in the departments of Rio Negro and Colonia in 2016 (EXP1: 33°02´S, 57°24´W and EXP2: 34°20´S, 57°55´W, respectively); two in the departments of Colonia and Paysandú in 2017 (EXP3: 33°49´S, 58°02´W and EXP4: 32°32´S, 57°48´W, respectively), and one in Paysandú during 2018 (EXP5: 32°32´S, 57°91´W). The experimental sites were selected based on confirmed Rcc presence in barley crops. Rcc was confirmed by sporulation on symptomatic barley leaves in moist chambers. These were observed by optical microscopy and it was possible to identify Rcc by typical morphology of conidiophores and mycelium arranged longitudinally along the leaf veins8.
Experimental cultivars encompassed Arcadia (EXP1, highly susceptible to RLS), INIA Arrayán (EXP4, moderately susceptible to susceptible to RLS), Musa 19 (EXP3 and EXP5, moderately susceptible to susceptible to RLS), and Danielle (EXP2, susceptible to RLS), as classified by Castro and others31. Crops were managed following standard agricultural practices in Uruguay22. Weather data including average temperature (°C), relative humidity (%), accumulated rainfall (mm) and rain events (number) during the crop growing cycle were obtained from records available at the experimental sites and/or nearby automatic weather stations in Dr. Mario A. Cassinoni Experimental Station, National Meteorology Institute, and National Agricultural Research Institute.
2.2 Experimental layout
The experimental design was a randomized complete block design with four replicates with 14 treatments. Twelve treatments were based on four fungicide mixtures: Abacus® + Zeta®, Cripton®, Xantho®, and Reflex Xtra® (Table 1, a) and three application moments32: GS33, GS47 and GS33+47 (Table 1, b). In addition, two treatments were included as controls: untreated (without fungicides, T1), and fully protected (T14), that consisted of three applications of Xantho® at GS33, GS47, and GS61. The experimental units were 3 × 5 m. All assessments were carried out in the four central rows to avoid border effects.
Fungicide treatments were applied using a CO2 pressurized backpack sprayer (D201 S, Spraytec, Argentina), equipped with four flat fan nozzles (TeeJet 11002, TEEJET, United States), at 300 kPa pressure, delivering a volume of 115 L ha-1. Fungicide doses corresponded to label recommendations.
Table 1: A. Commercial fungicides used in this study, including active ingredients concentrations’ application rates and pre-harvest interval
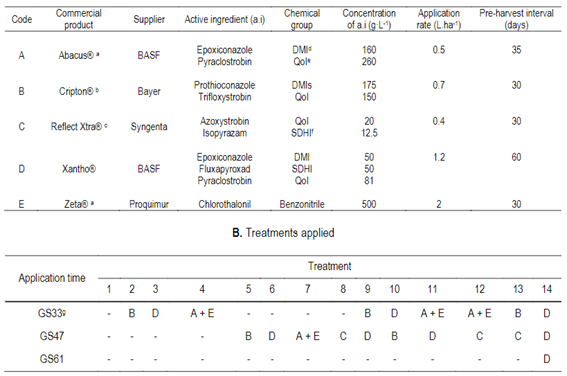
aused with Dash (37.5 g, methyl oleate and methyl palmitate); bused with Optimizer (76.5 g, methylated vegetable oil); cused with Nimbus (42.8 g, paraffinic mineral oil); dDMI: demethylation inhibitors, eQoI: the quinone outside inhibitors; fSDHI: succinate dehydrogenase inhibitors; gGrowth stage (GS), Zadoksand others32.
2.3 Fungicides and application times
Fungicides were selected based on their high efficiency to control RLS, previous field experiments across years and locations, and their commercial use and availability in Uruguay 5)(16) 33. The timing of fungicide application was determined by considering different potential types of RLS epidemics: at stem elongation (GS32-38) as a response to early disease onset, at awns-peeping (GS47-49) for a late disease onset, and at both application times for continuous conductive conditions for RLS development throughout the crop growth cycle10, under the assumption that the conditions for the development of RLS epidemics were optimal and that the inoculum of Rcc was not limiting. It should be worth noting that recommendations for fungicide applications in Uruguay consider other variables in addition to the crop growth stage, such as environmental conditions, inoculum pressure, disease onset, and crop potential6.
2.4 RLS control efficiency and plump grain yield
Ramularia leaf spot severity was determined for each plot and was expressed as the mean value for all leaves evaluated in 10 main stems, which were randomly selected from the central four rows. RLS assessments were performed prior to each fungicide application and then every 10-15 days depending on RLS pressure and development. Based on the disease severity values, the area under the disease progress curve (AUDPC) was estimated according to Campbell and Madden34. In addition, control efficiency (CE) was calculated based on AUDPC values35, as follows:
% CE = ((AUDPC untreated control- AUDPC treatment) / AUDPC untreated control) * 100.
Grain yield was determined by manually harvesting all spikes in the four central rows of each experimental plot, once the grain had reached its physiological maturity. Subsequently, grains were collected in paper bags, labeled accordingly by plot, and taken to the laboratory for moisture correction to 15% to determine grain yield and plumpness (percentage of grains > 2.5 mm) in C480988 Weg Motores SA (SC, Brazil). Plump grain yield (kg ha1) was estimated by the multiplication of grain yield (kg ha-1) and grain plumpness. Samples were then kept in a freezer (Thermo Scientific, Asheville, NC, US) at -80 °C for the subsequent analysis of fungicide residues.
2.5 Analysis of fungicide residues in barley grain
Field experiments were designed to meet the constraints of the GAPs, while considering the highest likely residue levels. Since repeated and late applications during the crop cycle most probably affect pesticide residue content in the grain21, grain samples from treatments with double application (GS33 and GS47) were selected to evaluate fungicide residues (T9, T10, T11, T12, and T13). These treatments would allow the evaluation of fungicide residues from both applications moments, GS33 and GS47.
Residue experiments should reflect the proposed critical GAPs (number of applications, interval between applications) to cover the worst-case residue scenario23. Therefore, the triple application was included in the evaluation (T14). However, the third application at GS61 is not recommended as it would not comply with the pre-harvest interval (60 days) indicated on the Xantho® label. It should be noted that all other applications evaluated in this study complied with the labelled pre-harvest intervals.
Hence, we evaluated six treatments totalizing twenty-four samples in four replicates in each experiment. From the field sample of each plot, a total of 150 g of grain were ground in an IKA®-WERKE Model M20 (Wilmington, USA). Samples were analyzed according to the QuEChERS method reported by Palladino and others36. The analysis of epoxiconazole, fluxapyroxad, isopyrazam, pyraclostrobin, trifloxystrobin, azoxystrobin, and prothioconazole was done by a hybrid Triple Quadrupole-Linear Ion Trap-Mass Spectrometer (4000 QTRAP® LC/MS-MS, SCIEX Instruments, Foster City, CA, USA). Chlorothalonil was analyzed by Agilent Technologies (Santa Clara, CA) 7890 with a 5977B MS system equipped with an HP5-MS column (30 × 0.25 mm i.d. × 0.25 μm) and operated under NCI mode. The instrumental parameters and the limits of quantification used were based on Palladino and others36.
Data were analyzed by frequency of samples testing positive, which was calculated as the absolute frequency of fungicide residue detections and quantifications over the total number of samples evaluated. This was calculated using 24 samples for each experiment, and 20 samples for the double and triple treatments.
The persistence of residues on or in the plant is influenced by the physicochemical properties of the active ingredient23. For this reason, the detection frequencies of fungicide residues were compared with their physicochemical properties (Table 2). Moreover, the values of fungicide residues were compared with the MLR settled by the European Union24 and the Codex Alimentarius25.
Table 2: Fungicide properties, limits of quantification, and Maximum Residue Limits (MRLs) established by the European Union (EU) and Codex Alimentarius for each of the active ingredients studied.
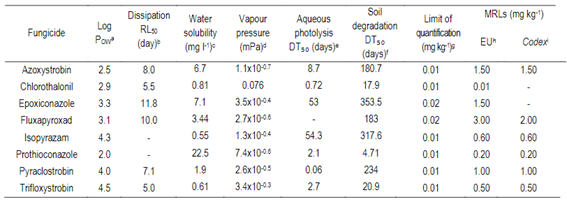
a Log POW: Octanol-water partition coefficient at pH 7.20 °C37; b Dissipation rate residual lifetime (RL50) of the pesticide on the specified plant matrix37; c Solubility - In water at 20 °C (mg l⁻¹)37; d Vapour pressure at 20 °C (mPa)37; e Aqueous photolysis DT₅₀ (days) at pH 737; f Soil degradation DT₅₀ in the field (days)37; g Limit of quantification36; h European Union24; i Codex Alimentarius25.
2.6. Statistical analysis
Data were analyzed separately by field experiment due to differences in weather conditions, RLS onset and severity. Statistical analysis was conducted using InfoStat® software v. 2020I38. RLS severity data in the untreated control was transformed to fit the logarithmic model in order to estimate disease progression from each experiment. Thus, Log Y_RLS = a + bx, x = days, a = the intercept of the line, and b = rate of disease increase (slope of the line).
Analysis of variance (ANOVA) was used in the general linear models (GLM), where the F statistics were significant (P< 0.01). Tukey’s protected least significant differences at α = 0.05 were used to determine the significance difference between treatments mean. ANOVA was used to evaluate the effect of different fungicide treatments on AUDP, CE, and plump grain yield of EXP1, EXP3, and EXP5.
In EXP5, Pearson correlation coefficient (r) and /or regression analysis were used to evaluate the relationship among RLS AUDPC and plump grain yield; the application rate, and concentration of fungicide residues. In addition, differences in RLS severity in the flag leaf of GS83 were tested using orthogonal contrast.
3. Results
3.1 RLS development
RLS epidemics developed differentially among experiments (Figure 1, a). Weather conditions at the beginning of the crop cycle (sowing to GS33) in terms of total rainfall (230-323 mm), rainfall events (18-53 days), temperature (11-14 °C), and relative humidity (80-87%) were conducive to RLS development across all experiments (Figure 1, b). However, disparities between the experiments became evident from GS33 until harvest. EXP1 and EXP5 displayed the lowest total rainfall amounts compared to EXP2 and EXP4 (43 mm, 119 mm, 196 mm, and 259 mm, respectively). Conversely, EXP3 recorded the highest total rainfall (532 mm) and the greatest number of rainy days (37 days). However, the average temperature increased in all cases, reaching an average of 18 °C.
Ramularia collo-cygni was detected in all five experiments; however, due to poor RLS development in EXP2 and EXP4, these were discarded from further analysis. An early onset of RLS was observed in EXP1, reaching 8% of severity in GS33 with a disease rate of 0.052 in the untreated control. Regarding EXP3 and EXP5, RLS developed late in the growing cycle and showed mean severity levels of 5% and 9% in GS47, respectively. The disease rate in both epidemics was similar: 0.087% and 0.078% of RLS severity, respectively. However, the time to disease development from GS33 to GS83 was shorter in EXP3 than in EXP5 (43 and 58 days, respectively). This resulted in a lower level of AUDPC in the untreated control in EXP3. Independently of RLS epidemics, the highest severity values were observed soon after GS47. The mean severity ranged 70-100% in GS83, with a maximum in EXP1 (96%), while it was similar in EXP3 and EXP5 (83% and 85%, respectively).
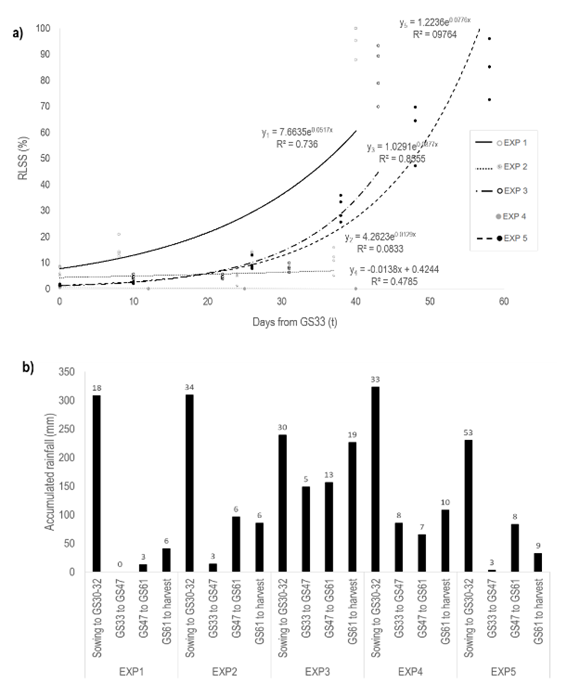
Figure 1: a Evolution of Ramularia leaf spot severity (RLSS) in the untreated control in each experiment: EXP1: Río Negro 2016, EXP2: Colonia 2016, EXP3: Paysandú 2017, EXP4: Colonia 2017, and EXP5: Paysandú 2018. b Accumulated rainfall and rainy days (values on accumulated rainfall bars, rains > 1 mm) registered in four crop phenological periods: i) from sowing to stem elongation (GS30-32), ii) from stem elongation (GS33) to first awns visible (GS47), iii) from GS47 to first anthers visible (GS61), and iv) GS61 to harvest
3.2 Effect of fungicides on AUDPC RLS
Only in EXP5, all fungicide treatments significantly reduced AUDPC values compared to the untreated control (ANOVA, p <0.0001). In EXP1 and EXP3, treatments were able to reduce AUDPC depending on the application time and the fungicide mixture used (Table 3). The late RLS epidemic was observed in EXP3, where only the double treatments (T10 and T13) with epoziconazole + fluxapyroxad + pyraclostrobin or azoxystrobin + isopyrazam at GS33 and prothioconazole + trifloxystrobin at GS47 were able to reduce severity to the same extent as the fully protected treatment (ANOVA, p < 0.0001). In contrast, in the case of EXP1, where an early development of RLS occurred, fungicides applied both at GS47 and at GS33 and GS47 reduced RLS severity regardless of the fungicide mixture used (ANOVA, p < 0.0001).
Table 3: Effect of different fungicide treatments on the area under the disease progress curve (AUDPC) of Ramularia leaf spot (RLS), control efficiency (CE), and plump grain yield of EXP1, EXP3, and EXP5
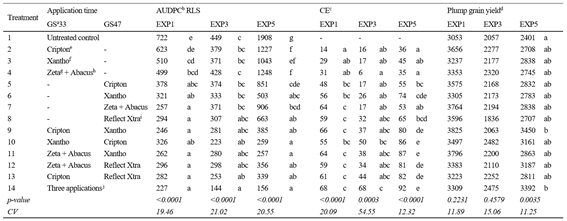
aGrowth stage (GS), Zadoks and others32; bArea under disease progress curve (AUDPC) values were calculated according to Campbell and Madden34; cCE was calculated based on AUDPCs values according to Abbott35; dPlump grain yield (kg ha-1) was estimated by the multiplication of grain yield values by grain plumpness (percentage weight of grains retained over a 2.5 mm sieve); eCripton (prothioconazole + trifloxystrobin); fXantho (epoxiconazole + pyraclostrobin + fluxapyroxad); gZeta (chlorothalonil); hAbacus (pyraclostrobin + epoxiconazole); iReflect Xtra (isopyrazam + azoxystrobin) jXantho sprayed at GS33, GS47 and GS61. Means followed by different letters in columns are significantly different according to Tukey’s HD test at p<0.05; CV: coefficient of variation (%).
3.3 Effect of fungicides on RLS control efficiency
Fungicide treatments provided CE ranges from 14 to 68% in EXP1, 5 to 68% in EXP3, and 35 to 92% in EXP5 (Table 3). Despite these variations and differences in RLS epidemic, the CEs of the treatments applied at GS33 were low (<45%). On the other hand, treatments with double applications had similar CE compared to the three applications treatment (T9-T13 vs. T14). In the early epidemic (EXP1), treatments applied at GS47 had similar CE to double applications and the fully protected treatment. However, in late epidemics at the same time of application, the CE differs depending on the fungicide. Isopyrazam + azoxystrobin (T8) in EXP3 and fluxapyroxad + epoxiconazole + pyraclostrobin (T6) in EXP5 applied at GS47 showed high CE values, similar to the fully protected treatment (Table 3).
3.4 Effect of fungicides on plump grain yield
Significant differences in plump grain yields were observed among treatments in EXP5 (ANOVA, p = 0.0035, Table 3). The only treatment with significantly higher plump grain yield than the untreated control was prothioconazole + trifloxystrobin at GS33 and epoxiconazole + pyraclostrobin + fluxapyroxad at GS47 (T9). All other treatments did not significantly increase plump grain yield in response to fungicide application.
Plump grain yield showed a negative and moderate correlation with the RLS AUDPC in EXP5 (Pearson r = −0.50, p = 0.0001). However, regression analysis results from EXP5 revealed that RLS AUDPC had a significant relationship with plump grain yields (y = -0,6431x + 2591,5, R² = 0,2518; p < 0.001). AUDPC contributed 25% to the observed variation in plump grain yield.
When analyzing the remaining green tissue amount at GS83 in EXP5, expressed as the number of live leaves minus the percentage of diseased tissue (severity), differences between treatments were observed (ANOVA, p < 0.0001). The treatments applied at GS33 had 0.27 mean green tissue and did not differ from the untreated control, 0.2 mean green tissue. The double applications and treatments T5 (chlorothalonil + pyraclostrobin + epoxiconazole) showed the same amount of live tissue as the fully protected treatment (2.2 mean green tissue). In line with this, when only the severity of the flag leaf (mean 19.5%) was analyzed at the same phenological stage, some differences between treatments were observed (ANOVA, p = 0.0184). When the timing of applications was statistically contrasted, GS33 applications had a higher level of flag leaf severity than GS47 applications (contrasted, 31.43, p = 0.0056). In addition, GS33 and GS47 applications had a higher level of severity than double applications (contrasted, 32.60, p = 0.0007).
3.5 Fungicide residues in barley grain
The number of samples with fungicide residues at or above the limit of quantification differed among experiments. EXP1 had the highest detection frequency, with 71% of the evaluated samples showing residues, whereas no residues were detected in EXP3. The other experiments showed a variation in the percentage of fungicide residues detected (EXP4 42% > EXP5 33% > EXP2 25%). There was no clear relationship between the percentage of detection in the northern (EXP1, EXP3, and EXP5) and southern (EXP2 and EXP4) production areas of the country, or among the different growing seasons studied (2016, 2017, and 2018). However, the percentage of residues detected in grains was influenced by weather conditions. There was a negative relationship between accumulated rainfall and rainy days in each experiment and the percentage of residue detected. The experiments with the most contrasting in terms of fungicide concentration were EXP1 and EXP3. Despite the fact that EXP3 had the highest accumulative rainfall (531 mm on 37 rainy days) from GS33 to harvest, no fungicide residues were detected. In addition, at EXP3 rainfall occurred on the fourth and fifth days after applications at GS33 (7 mm and 11 mm, respectively), and 42 mm on the fifth day after applications at GS47. On the contrary, during the same period, EXP1, which had the lowest accumulated rainfall (43 mm on 8 rainy days), presented the highest number of fungicide detections in the grain.
In all cases, the highest percentage of fungicide residues detected was associated with applications at GS47 (Figure 2, a). Only trifloxystrobin was detected in barley grains that received a single application at GS33, whereas applications at GS47 resulted in the detection of azoxystrobin, epoxiconazole, fluxapyroxad, isopyrazam, and pyraclostrobin. Interestingly, prothioconazole and chlorothalonil were not detected on barley grains in any of the samples.
EXP1, EXP2, EXP3, EXP4, and EXP5 showed different durations of the period from last application to harvest (43, 44, 53, 53, 48, and 46 days, respectively). Furthermore, they did not show a clear relationship with the percentage of detection of residues found at T14 (75, 75, 0, 42, and 17%, respectively).
When analyzing the results from the triple applications (T14) separately by fungicide, fluxapyroxad was detected in the range 0.020-0.578 mg kg-1 in all experiments. Pyraclostrobin was detected in three experiments, EXP1, EXP2, and EXP4, in the range 0.028-0.227 mg kg-1. Epoxiconazole was only detected in EXP1 and EXP2 in the range 0.082-0.148 mg kg-1. Only in EXP1 and EXP2 all fungicides were detected and with the highest detection frequency. This may be related to the lowest total rainfall and rainy days from GS61 to harvest in EXP1. However, in the other experiments, there was no clear relationship between rainfall or rain events and the percentage or concentration of residue detections (Figure 1, b).
The application rate of each active ingredient had no direct relationship with the concentration of fungicide residues found (Pearson r: -0.07, p-value = 0.1579). For example, chlorothalonil had the highest rate of application whereas was not detected in the grains. Finally, the fungicides doses and timing of applications that were used in this study resulted in residue concentrations below the MRLs established by the European Union22 and the Codex Alimentarius23 (Figure 2, b; Table 2).
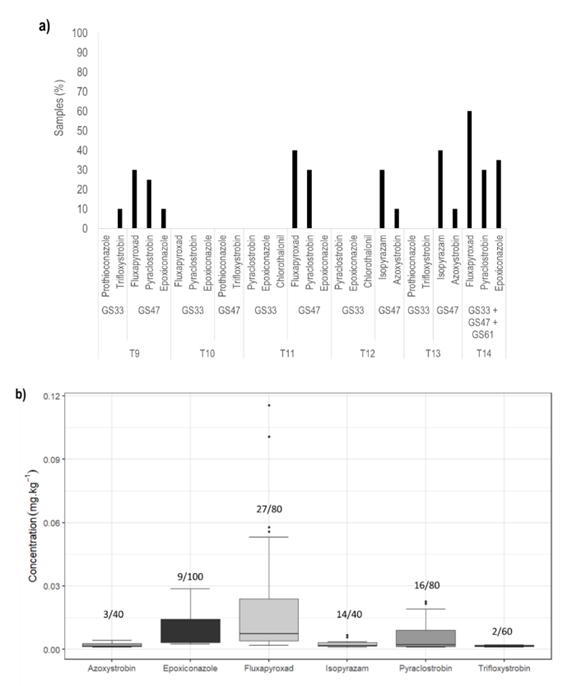
Figure 2: a. Percentage of frequency of samples with fungicide residues detected at or above the limits of quantification37 in treatments with double or triple application of fungicides in all experiments. Frequency was estimated based on the analysis of 20 samples per treatment. b. The concentration of fungicide residues detected was expressed in mg kg-1 for each of the active ingredients studied. The values in the bars represent the number of samples at or above the limit of quantification37 out of the total number of samples that could have contained that fungicide
4. Discussion
Results from this study revealed that all fungicide management strategies evaluated to control RLS of barley complied with the MRLs as established by Codex Alimentarius25 and the European Union24. This work involved strategies based on the most effective fungicides for RLS control used for the different types of RLS epidemics registered under Uruguayan conditions: early and late onset, and early, continued epidemic throughout the crop growing cycle, where environmental conditions were highly favorable for RLS development. The results showed that the efficiency of the fungicides to control RLS was conditioned by the disease development, the time, and the number of applications, and this agrees with vast information from Europe5, Argentina16, and Uruguay6. All the fungicide mixtures tested at GS33 + GS47 were effective in controlling RLS under the assayed conditions. However, at GS33 or GS47, the efficacy of the mixtures depended on the epidemic type.
4.1 Effect of fungicides on AUDPC RLS, CE, and plump grain yield
Ramularia collo-cygni was detected in all the experiments at early crop stages (GS33), but RLS did not develop further in EXP2 and EXP4. Rcc behaves as an endophyte and may become a pathogen causing symptoms under certain stress 8)(39) . In addition, in UK and Poland some studies reported that detected airborne Rcc in areas where symptoms are rarely observed40. For this reason, the detection of Rcc in the crop does not necessarily indicate an epidemic.
Once Rcc has been identified, the crop should be fully monitored, taking into account climatic conditions. In EXP2 and EXP4, environmental conditions from GS33 to GS47 were not conducive to epidemics of RLS. Furthermore, no significant crop stress factors were recorded during this period, such as scarce water availability, sequence of wet and dry days, high light intensity, waterlogging, nutrient deficiency, and prolonged leaf-wetness duration 7)(8) 39 that might have triggered RLS development. Moreover, the barley varieties used in the field experiments (Musa 19, and Danielle) had the lowest susceptibility to Rcc compared to the other varieties tested in this study. Interactions between the genotype of the barley varieties39 and environmental conditions 7)(8) 39 appear to play an important role in the expression of RLS.
In EXP1, EXP3, and EXP5 the onset of RLS epidemics differed, with earlier development in EXP1 than in the others. This could be due to differences on cultivars’ susceptibility, which are highly susceptible in EXP1 and moderately susceptible in the other experiments. The high conduciveness of weather conditions for RLS after GS33 in the experiments might have resulted in high RLS severity at the end of the cycle. Adverse environmental conditions, such as prolonged drought or heavy rains, accentuate the interactions between hosts-pathogens 41)(42) . From a physiological point of view, abiotically stressed plants are in an unbalanced state, which could affect host resistance, leading to an increase in epidemic severity 7)(42) . Moreover, in EXP1 and EXP5, water deficiency during a critical period in the crop could have further enhanced the effects of RLS 3)(5) 39. According to other reports, warm and dry conditions suggest that dew formation and single rain events may still have been sufficient for significant outbreaks of RLS7. On the other hand, in EXP3 the variable pattern of rainfall after flowering may be related to an increase in RLS severity43.
In EXP1, EXP3 and EXP5, the typical symptoms of RLS were observed after GS47, in agreement with Pereyra and Pérez6, who reported the detection of typical symptoms before GS51 in those years favorable for RLS. In Europe, typical symptoms are usually developed in the crop after spike emergence3. However, under conducive weather conditions, symptoms can be detected even earlier. Diagnosis of RLS, before the typical symptoms are observed, is very difficult, as the spots are confused with symptoms of other leaf spot pathogens or biotic agents5. Therefore, correct detection is essential for management. A nice strategy could be identification of Rcc by microscopy, but requires trained personnel to select the correct technique and leaf material. Also, it can only be performed when symptoms are visible in barley leaves14 or implementing the wet chamber incubation. Despite the training of technicians, wet chamber incubation does not provide accurate diagnosis, being the most efficient techniques for detection and identification of Rcc the PCR techniques13.
Regarding CE in early or late epidemics, treatments with a single application at GS33 did not control RLS significantly, because the disease outbreak occurred later in the season (after GS47). The CE in applications at GS33 might have been conditioned by the limited period of action of the treatments. Moreover, in the case of an early epidemic, the CE was also conditioned by the high severity of RLS at the application moment.
In the early epidemic, fungicide treatments including applications at GS47 (single GS47 and double GS33+GS47) were efficient for RLS control without the need for a third application (fully protected), although weather conditions remained favorable for RLS after GS47. Applications after the recommended application window (GS31-GS49) did not show an acceptable CE and are considered late applications for RLS control6.
On the other hand, in the late epidemics, CE at GS47 was dependent on the fungicide used, and better control was achieved using mixtures containing a SDHI (fluxapyroxad or isopyrazam). This is aligned with the literature that mentions that SDHI is one of the most efficient fungicide groups for RLS control5. However, treatments with double applications (GS33+GS47) were the most efficient, probably due to the disease development under the studied conditions that appeared at the end of the barley cycle. However, the double application treatment did not differ from the fully protected treatment in terms of RLS control. This underlines the importance of applying on time, as this improves efficiency, reducing the number of applications.
Resistance or decreases in sensitivity of Rcc are being reported worldwide to the DMI´s, SDHI´s 16)(18) 19, and QoIs fungicide groups 6)(16) 20. Although the sensitivity of the DMI´s and SDHI´s groups was not assessed in this study, the fungicide mixtures containing these types of fungicides were efficient against RLS under the studied conditions.
Regarding plump grain yield, there were no differences in plump grain yield except in EXP5, with the latest and longest RLS epidemic. A negative correlation between plump grain yield and RLS AUDPC was observed in EXP5. However, the regression model indicates that losses in plump grain yield may not only be correlated directly with the level of RLS. Plant health was not the main limiting factor in achieving yield, as only RLS was detected in the crop. Despite the fact that the amount of green tissue remaining in the plant at the end of the growing cycle (GS83) differed between treatments and in the flag leaf, no differences in grain yield were observed. This may be due to the fact that the time at which epidemic RLS levels were recorded in all experiments coincided with the critical stage for determining the final grain yield.
4.2 Fungicide residues in barley grain
As mentioned above, all the fungicide management strategies evaluated to control RLS, even the one that aimed a fully protected treatment (T14), complied with the MRLs set by Codex Alimentarius25 and the European Union24. These results were consistent with those observed in our previous report, in which the residues of the most commonly used fungicides to control RLS in barley grains collected from commercial fields in 2017 were evaluated30.
The presence of fungicide residues in the grain obtained in the experiments can be attributed to a combination of factors, such as weather conditions (e. g., air temperature, relative humidity, sunlight intensity, accumulated rainfall, rainy days), spray application technology (e. g., droplet size, volume rate, ground speed), fungicide rates, physicochemical properties of the fungicides (e. g., Log POW, dissipation rates on plant, vapor pressure, water solubility, soil degradation), and the compliance with pre-harvest interval recommendations 29)(44) 45. These interrelated factors contribute to the complex landscape of the fungicide residues found in the evaluated treatments.
In this research, fungicide residues in grain were conditioned by the different experimental environments and not by locations or growing seasons. The number of rainy days and the accumulated rainfall in each experiment could have influenced the frequency or the concentration level of each fungicide residue in the harvested grain. Interestingly, a greater amount of total rainfall and rainy days from GS33 to harvest correlated with fewer residues detected in the grain. Weather conditions significantly impact residue behavior in and on plants, especially immediately after application 23)(46) .
Another factor affecting the residue frequency in grains was the period of time between the application and harvest, notably at growth stages GS47 and GS61. Late applications had less time for degradation compared to GS33, leading to the presence of residues in the grain. Applications at GS61 exceeded the 60-day pre-harvest interval specified on the Xantho® label, resulting in anticipated grain residues. This emphasizes the importance of following the label recommendations. The results obtained highlight the necessity for local studies to establish national pre-harvest intervals. The period from the last application to harvest and the environmental conditions such as accumulated rainfall, rainy days, and timing of rainfall may have influenced the presence and concentration of residues. Rain is considered the biggest detractor of fungicide deposits on the leaf surface47.
The application rate of each active ingredient is different, although no direct relationship with its concentration was found in grains. Fungicides’ detection and concentration might be conditioned by their physicochemical properties. For instance, the dissipation rate lifetime (RL50) on and in the plant is an additional parameter that provides valuable information to explain the degradation of pesticide residues37. Accordingly, we found that fungicides with higher RL50 were more frequently detected in grains than those with lower RL50.
Trifloxystrobin was only detected at GS33 in EXP2 and EXP5, which may be due to limited rain post-application (GS33 to GS47). Its absence in residues after GS47 application could be attributed to its high vapor pressure, leading to volatilization. In addition, an increase in average temperature from GS47 to harvest across all trials contributed to pesticide disappearance from plants through its influence on pesticide vapor pressure and volatility 46)(48) . In addition, trifloxystrobin is considered non-persistent in soil.
The log POW, known as the octanol-water partition coefficient37, informs about the lipophilicity of molecules, and is commonly used to explain the presence of pesticide residues in certain products. Our results showed that all fungicides with log POW> 2 were detected in grains, except chlorothalonil, which was not detected in this study. Although they are detected at low-level concentrations, what happens to these fungicides in malt should be investigated. Several studies 25)(49) 50 mention that pesticides with log POW values > 2 may remain on malt.
On the other hand, prothiconazole presented log POW values = 2, also one of the most water-soluble fungicides in this study and not persistent in soil, which may have contributed to the non-detection of this active ingredient in grains in this study.
For chlorothalonil, the absence of residues in the samples could be explained by several factors. Firstly, this fungicide was applied at early stages of the crop (GS33) and complied with the pre-harvest interval established on the fungicide label. Applications at GS33 had a longer period from application to harvest than applications at GS47, thus decreasing the probability of detecting residues in grains. Moreover, chlorothalonil is a contact fungicide that may remain on the plant surface and thus undergo several biochemical processes such as photodegradation and volatilization that may lead to its dissipation in the environment 51)(52) . From the selected fungicides, chlorothalonil has the fastest photodegradation, a few days of dissipation RL50, and the highest vapor pressure. These events may result in a reduced likelihood of chlorothalonil presence in the harvested grain.
5. Conclusion
These results confirm that the main fungicide management strategies recommended for RLS control in Uruguay are aligned with both national and international regulations. However, the efficacy of the evaluated fungicide mixtures was found to be influenced by several factors such as RLS development, timing, and number of applications. This highlights the lack of a single approach to control and the need for adaptability on a case-by-case basis. Particularly, the choice of an RLS management strategy from the proposed options is based solely on its efficacy, as it poses no risks in terms of the presence of fungicide residues in barley grain.
Based on the results of this study, it can be assumed that the fungicides tested would not be transferred to the wort and consequently to the beer, as they comply with internationally recognized MRLs. However, if there are changes in fungicide management practices, it is advisable to re-evaluate fungicide residues in grain. Furthermore, it is crucial to understand that MRLs are subjected to continuous assessment by global organizations, thereby having the potential to change. In the event that the MRLs of certain fungicides are lowered, stakeholders could encounter marketing challenges due to non-compliance concerns.
The complexity of the interactions between the presence of fungicide residues in grains, the environmental conditions, the timing of applications and the physicochemical properties of the active ingredients highlights the importance for local studies to establish pre-harvest intervals at national level.