Introduction
Peach and nectarine production in Uruguay is of around 12,858 and 1,550 tons, which covers an area of 1,388 and 160 ha, respectively, exploited by 600 producers and destined 97 % to the domestic market, 2.8 % to industry and 0.2 % for export1.
Both fruits are of the climacteric type, which determines their fast deterioration after harvest if they are kept at room temperature (15-20 °C), due to the increase in the respiratory rate and ethylene production (C2H4). Therefore, cold storage has been used as a tool to extend shelf life2)(3. It has been shown that the optimum storage temperature is 0 °C, while relative humidity should be between 95 and 98 %. Under these conditions, the respiratory rate is between 2-3 mL of CO2 kg-1h-1 and the C2H4 production between 0.5-5 µL kg-1h-1.
On the other hand, peach and nectarine fruit are sensitive to cold, so storage at non-optimal temperatures, between 2 and 8 °C, can cause physiological disorders called «chilling injury»4. Symptoms of chilling injury (ci) include internal browning, flesh mealiness, redness or bleeding, flesh translucency, inability to ripen, among others5 which are expressed when the fruits are transferred to room temperature (shelf life, sl), even though they occur during refrigerated storage. Therefore, the problem does not arise until the fruit reaches consumers, which limits the sl and leads to a reduction in consumer acceptance and, consequently, a value loss6. The susceptibility degree has a strong genetic component, which indicates that in general peaches are more sensitive to developing ci than nectarines4)(7. In the case of Big Top, Candir and others8 indicate that they have a conservation potential of 30 days at 0 °C, followed by 2 days of sl at 20 °C.
From the physiological point of view, the first ci effect occurs at the cell membrane level that passes from a crystalline-liquid state to a gel structure, affecting its selective permeability, which causes the leakage of cytoplasmic content, ions, and metabolites9)(10. An indicator of membrane stability is the malondialdehyde (mda) content, which is known as the final product of lipid peroxidation that constitutes it. As a consequence of this permeability loss, the enzyme polyphenol oxidase (ppo) and polyphenols also come into contact, which in the presence of oxygen (O2) form dark pigments responsible for browning, one of the ci symptoms11.
Another of the ci symptoms is flesh mealiness, related to an imbalance between the activity of enzymes involved in cell wall degradation, pectinmethylesterase (pme) and polygalacturonase (pg)12)(13)(14.
During storage under inductive temperature conditions, reactive oxygen species (ros) are generated, such as superoxide (O2-), hydroxyl (OH-) and hydrogen peroxide (H2O2), which accumulate when there is an imbalance between its production and depletion due to failure in the fruit’s antioxidant system. ros production also contributes to membrane damage15)(16. The antioxidant system consists of enzymes that include superoxide dismutase (sod), catalase (cat), ascorbate peroxidase (apx) and glutathione reductase17. These enzymes act as direct antioxidants, which eliminate O2- and H2O218. sod catalyzes the dismutation of O2- to H2O2 and O2, while cat and apx catalyze the decomposition of H2O2 in O2 and H2O19. In addition to enzymes, there is a non-enzymatic antioxidant system that comprises a broad group of substances that can act as receptors or metal ion chelators, and include total polyphenols, ascorbic acid, flavonoids, and others. The high antioxidant activity of these compounds is mainly due to their potent reducing capacity, oxidizing preferentially in the presence of oxidizing substances and thus protecting the cellular structures20.
There are different treatments used to mitigate the occurrence of cold damage, which may be chemical, such as the use of 1-methylcyclopropoen (1-mcp), salicylic and jasmonic acid, among others, and/or physical, such as controlled or modified atmospheres, uv-c radiation and heat treatments21)(22. Postharvest heat treatments can be applied as hot water (40-60 °C for 7-10 min), hot air (30-40 °C for 24 to 48 h) or steam3. The reduction of the ci incidence in thermally treated fruit could be attributed to the improvement of the integrity of the membrane, to the expression of the genes encoding the heat shock proteins and/or to the improvement of the antioxidant system activity, among others23.
This study aimed to evaluate the effect of hot air treatment on metabolic activity and the enzymatic and non-enzymatic antioxidant systems of nectarine fruit, Big Top variety, preserved at two temperatures, one considered optimal (0 °C) and another considered stressful (5 °C).
Material and Methods
Plant material and heat treatment
The Big Top nectarine variety, harvested on December 20 on a commercial property in Melilla (Montevideo, Uruguay), was used. The fruit presented an average weight of 125 g. The harvest time was defined based on the background color, the total soluble solids (°Brix) and the flesh firmness. Fruit were harvested with a yellow-greenish color, 14 °Brix and an average firmness of 45 N measured in the equatorial section of the fruit.
Immediately after harvest, the fruit were transferred to the Fruits and Vegetables Postharvest Laboratory of the Agronomy School, located approximately 15 km away. Once in the laboratory, they were selected and placed in plastic boxes (seven fruits per box) and subsequently divided into four groups, two of which were placed in a closed enclosure of 3.5 x 2.5 m, heated at 35 ± 3 °C for 36 h, with temperature monitoring. The groups that did not receive heat treatment were immediately stored in refrigerated chambers at 0±1 and 5±1 °C, respectively, with 95% rh. After the heat treatment, the fruits were divided into two groups and also stored at 0±1 and 5±1 °C together with the untreated ones. After 6, 18 and 27 days of conservation, 3 boxes per treatment were taken (21 fruits in total) for the analyzes corresponding to the end of conservation, while another 3 boxes were left for 6 days at 20 °C to carry out evaluations corresponding to sl.
Respiratory rate
For the determination, two fruits were placed in glass jars provided with an airtight lid and a silicone septum. After 2 h, 10 mL of gas were extracted using a syringe, and injected into a gas chromatograph (Agilent Technologies 7890B, cg System, United States), with an injector, oven and detector temperature of 20, 60 and 200 °C, respectively. Three repetitions were performed per treatment (treated and control fruit) and by storage temperature (0 and 5 °C), and the values were expressed as mg CO2 kg-1h-1.
Weight loss
The fruits were weighed at harvest (initial weight) and at each moment of analysis, both in conservation and sl, using a digital balance (Acculab, vi-10 kg, usa). Data were expressed as percentage of weight loss.
Total polyphenols
The total polyphenols (tp) were determined in 100 µL of extract obtained from 3 g of flesh from frozen samples (-80 °C), homogenized with 9 mL of methanol using a disperser (Ultraturrax xhd-d High-Speed Disperser, China) for 1 min at 13,500 rpm24. After that, 150 µL of the Folin-Ciocalteu reagent (1:1 with distilled water, Merck, Germany) were added to this extract, and after 3 min 1,000 µL of a 0.4 % sodium hydroxide solution (NaOH, MW: 39.99 g mol-1; Carlo Erba, Italy) and 2 % sodium carbonate (Na2CO3, pm: 105 g mol-1; Merck, Germany) were added. The absorbance was then measured by a uv-visible spectrophotometer (Unico, S-2150, usa) at 750 nm. Three repetitions per treatment were performed. The results were expressed in mg gallic acid equivalent in fresh weight (mg gae g-1 fw) with gallic acid as standard (mw: 170.12 g mol-1; Merck, China).
Total antioxidant capacity
For this, 25 µL of the same extract used for the tp were mixed with 675 µL of the dpph reagent (mw: 394.32 g mol-1; Sigma-Aldrich, Germany), which reacted for 1 h to measure absorbance at 515 nm25. Three repetitions per treatment were performed. The results were expressed in mg ascorbic acid equivalent (mg aae g-1 fw) since ascorbic acid (mw: 176.12 g mol-1; Merck, China) was used as a standard.
Malonildialdehyde content
The MDA content was determined from 3 g of tissue homogenized with 15 mL of 5 % (w/v) trichloroacetic acid (tca, Merck, Germany). It was then centrifuged at 13,000 rpm for 30 min26. 2 mL of supernatant were taken and 2 mL 67 % tca were added. It was subsequently heated at 100 °C for 30 min, cooled rapidly and centrifuged at 10,000 rpm for 10 min. The absorbance was measured at 532, 600 and 450 nm, respectively. Three repetitions per treatment were performed. mda content was calculated using the following formula:
Where: Vt, extract volume; Vr, reaction mixture volume; Vs, volume of extract contained in the reaction mixture; and m, mass.
Oxidative stress enzyme activity
For the extraction, 1 g of lyophilized flesh was homogenized with 0.2 g of polyvinylpyrrolidone (pvpp, Merck, Germany); 5 mL extraction buffer (tpk, 50 mM and pH 7.8); 0.1 mM ethylenediaminetetraacetic acid (edta); 5 mM L-cysteine; 0.2 % triton X-100 and 1 mM phenylmethylsulfonyl fluoride. Catalase activity (cat) was measured in a quartz cuvette with 50 µL of the enzyme extract and 1,450 µL of reaction buffer 50 mM potassium buffer and pH 7 with H2O2 10 mM, registering absorbance values for 5 min at 240 nm. Calculations were made based on the increase in absorbance of the linear part of the reaction curve. The activity was expressed as units of enzyme activity (ua mg-1 of protein).
In the case of ascorbate peroxidase (apx), 505 µL of the extract and 960 µL of reaction buffer (50 mM tpk and pH 7; 0.1 mM edta; 0.5 mM ascorbic acid and 1.54 mM H2O2) were used. Measurements were taken every 30 s at 290 nm until the absorbance was constant. The calculations were made based on the decrease in the absorbance of the linear part. The activity was expressed as UA mg-1 of protein.
For the determination of superoxide dismutase (sod), 2 g of lyophilized flesh were weighed and homogenized with 5 mL of extraction buffer (50 mM Tris-HCl and pH 7.5; magnesium chloride and 1 mM edta) for 1 min. The mixture was centrifuged at 3,000 rpm for 20 min at 4 °C.
Two identical cuvettes were prepared with 6 µL of extract with 351 µL of reaction buffer (50 mM phosphate buffer and pH 7.8; 13 mM methionine; 75 µm tetrazolium nitroblue chloride, and 2 µM riboflavin). One was kept in the dark, and the other was exposed to the light of a 15 W lamp 30 cm away for 15 min, and then the absorbance was measured at 560 nm. In all cases, three repetitions were performed per treatment.
To determine the specific activity of the enzymes, the protein concentration of each of the extracts was determined following the Bradford methodology27. For this, 50 µL of each enzyme extract were taken and placed in quartz cuvettes together with 2,500 µL of Bradford reagent (Sigma-Aldrich, usa). After 30 min the absorbance at 595 nm was measured. To determine the protein concentration, a standard curve was carried out for each enzyme with bovine serum albumin dissolved in the extraction buffer corresponding to each enzyme.
Activity of enzymes that degrade the cell wall
In the case of pme, 1 g of lyophilized fruit sample was weighed and homogenized with 10 mL of sodium chloride (NaCl, mw: 54.44 g mol-1; Merck, Germany), 1 M containing 10 g L-1 of polyvinylpyrrolidone (pvpp, Merck, Germany) and 1 mM cysteine. The homogenized were then stirred at 4 °C for 4 h and centrifuged at 10,000 rpm for 20 min.
The activity was determined in the mixture containing 600 µL of 0.15 % w/v pectin (70 % esterification degree), 150 µL of 0.01% bromothymol blue in 0.003 phosphate buffer (pH 7.5), 100 µL of water and 200 µL of extract from the supernatant. Then, the absorbance at 620 nm was measured and the enzymatic activity was expressed as ua g-1 of protein.
For the pg extraction, 1 g of lyophilized sample was weighed and homogenized with 10 mL of HAc-NaAc (50 mM pH 5.5), which contained 10 g L-1 of pvpp, cysteine 1 and 1 M NaCl. Then, the homogenates were stirred at 4 °C for 3 h and centrifuged at 10,000 rpm for 20 min. The collected supernatants were dialyzed against NaAc (50 mM and pH 5.5) for 12 h. The activity was determined by a reaction mixture containing HAc-NaAc (50 mM and pH 5.5), 400 µL of 0.15 % w/v polygalacturonic acid and 200 µL of enzyme extract. Samples were incubated at 40 °C for 30 min. Then, 200 µL aliquots were taken, adding 1,000 µL of 1 M sodium borate; 2-cyanoacetamide and placed in boiling water for 10 min. Once cold, absorbance at 295 nm was measured and the activity was expressed as ua g-1 of protein.
In all cases, three repetitions were performed per treatment.
Statistical Analysis
A completely randomized design (crd) with a factorial structure was used. First, the heat-treatment factors (treated and untreated) and conservation time at each of the temperatures considered were analyzed, and subsequently the temperature conservation factors (0 and 5 °C) and treatment. An analysis of variance at 5 %significance was performed using the INFOSTAT Professional statistical program (Universidad Nacional de Córdoba, Argentina).
Results and Discussion
Respiratory rate
The respiratory activity for fruit stored at 5 °C and 0 °C presented initial values of around 6 mg CO2 kg-1 h-1. However, after 20 days, when the respiratory peak occurred, the magnitude of respiration of the fruit at 0 °C was around 12mg CO2 kg-1 h-1, while in those stored at 5 °C it was 24mg CO2 kg-1 h-1 (Figure 1). This may be due not only to the fact that respiration is a function of temperature but also to the fact that at 5 °C the fruits are in a stressful situation, so their respiration could also increase. In other cold-sensitive products, such as the Micro-Tom tomato, this behavior was also observed, where respiration was higher after 28 days at 4 °C compared to when it was stored at a higher temperature28.
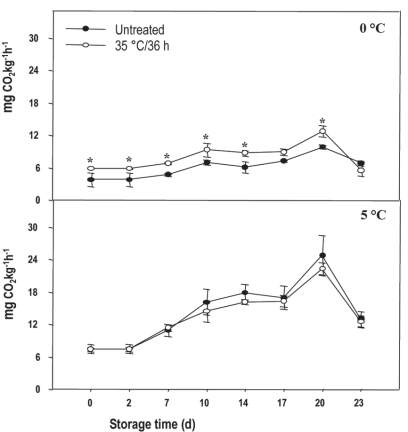
Figure 1: Respiratory rate in nectarines var. Big Top during storage at 0 and 5 °C. Values are means (n=3) ± standard error of the mean. The symbol (*) indicates significant differences for the treatment (Tukey p<0.05).
Considering the temperature of 0 °C, treated fruit showed in general between 2 and 5 % more respiratory activity than the untreated ones. This could mean that the heat treatment was too intense, determining heat stress that resulted in increased respiration. As found by other authors, heat treatments, both water and hot air (35-50 °C), at varying times, would inhibit the ripening processes of the fruit, reducing respiratory activity and the production of C2H4 in the postharvest period29, which was not observed in this study. On the other hand, no heat treatment effect was found when the fruit were stored at 5 °C. Therefore, the heat treatment was not effective in decreasing the respiratory activity of the Big Top peach fruit, as it did in the Hujimgmilu variety, when they were exposed to hot air (46 °C for 10, 20 and 30 min) before storage at 0 °C. In this case, the treatments managed to reduce the respiratory rate and offset the occurrence of the climacteric respiratory peak proportionally to the exposure time14.
According to Paull and Chenn30, fruit response to heat treatments, concerning quality preservation during storage, including the lower susceptibility to developing CI, is very variable and depends on several factors, such as exposure time, temperature, species, variety, maturity degree, fruit shape and size, among others.
Weight loss
Regardless of the storage temperature, weight loss increased with the storage course, reaching values between 14-20 % after 27 days and values between 15 and 25 % in sl (data not shown). These values are considered high since the visual quality of the fruit is reduced with values between 5 and 10 %31. Therefore, after 18 days there were already visible dehydration symptoms that noticeably affected the appearance of the fruit.
Fruit kept at 0 °C did not show a clear effect of the heat treatment since, after 18 days, the untreated lost 2 % more weight, an expected situation since the heat treatment would protect the membranes and reduce water loss. However, the effect changed and after 27 days the treated ones presented the highest dehydration (data not shown). However, in sl, the untreated fruit showed the greatest loss, 4 and 3 % more than in fruit subjected to heat treatment at 18+6 and 27+6 days, respectively, (data not shown). In the case of fruit kept at 5 °C, the untreated ones lost 2 % more weight than those treated at 18 and 27 days, while in sl there were no differences (data not shown).
According to different authors, heat treatments can cause increases or decreases in water loss, which will depend on their characteristics (method, temperature and exposure time), as well as the characteristics of the product. Heat exposure can damage the skin of the fruit, determining an increase in water loss due to any of the aforementioned factors, temperatures or excessive exposure times, which we believe was what happened in this case32.
Total polyphenols
According to the results of the heat treatment x time of storage analysis, in the fruit preserved at 0 °C, the values remained stable and around 0.24 mg gae g-1 fw without observing a heat treatment effect (Figure 2A).
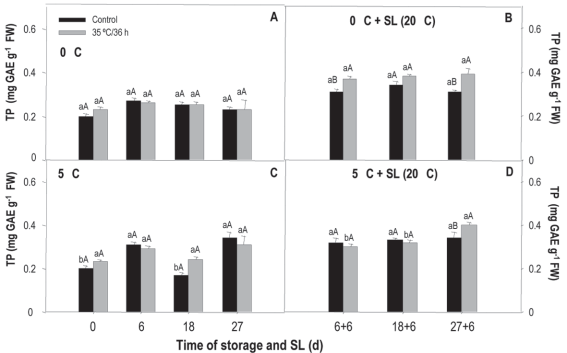
Figure 2: Total polyphenols in nectarine var. Big Top during storage at 0 and 5°C and in shelf life (sl). Values are means (n=3) ± standard error of the mean. Uppercase letters indicate differences for the treatment factor and lowercase for the time factor according to storage temperature (Tukey p<0.05).
However, in sl, the heat treatment determined an increase in the tp in the 6+6 and 27+6-day periods. When comparing the tp content during storage and sl, an increase was observed where the contents almost doubled, regardless of the treatment (Figure 2B).
Similarly, no differences were found in treated and untreated fruit preserved at 5 °C regarding the tp content, with initial and final values between 0.22 and 0.32 mg gae g-1 fw respectively (Figure 2C). At the end of the sl (27+6 days), the treated fruit presented 15 % more tp than the untreated ones (Figure 2D). In this case, the tp content did not show differences in the values registered during the storage and sl.
When analyzing temperature effect (0 and 5 °C) and heat treatment, no interaction or effect of the temperature was found. Only one heat treatment effect was observed that determined a higher tp content with values of 0.28 and 0.26 mg gae g-1 fw. Regarding the evolution of tp over time of cold-sensitive fruit stored at inappropriate temperatures, these present a progressive increase during the first week, considered as an alarm stage, when, according to reports, production of ros and antioxidants as a mechanism to relieve stress increases33 This behavior was observed in the untreated Big Top fruit at 5 °C, a situation considered favorable for ci development. The increase in tp content observed in sl compared to storage may be linked to the fact that the increase in temperature accelerates metabolism and more damage is revealed. This situation also activates the fruit mechanisms to a greater extent to protect itself against damage, which in this case implies an increase in tp.
Similar results were observed in the study conducted by Huan and others34, where tp in peaches treated with heat (hot air 38 °C/3 h) before cold storage increased considerably in relation to levels measured in untreated fruit. According to Spadoni and others35, the synthesis of tp was favored in peaches treated with hot water (60 °C/20 s), a process linked to the activation of the enzymes responsible for their synthesis.
In cucumbers treated with hot water (45-55 °C/5 min), tp levels increased both in storage at 4 °C (7 and 21 days) and, mainly, in sl at 20 °C (7+4 and 21+4 days). The authors attribute this behavior not only to the synthesis but mainly to the reduction of the activity of the enzymes involved in their degradation, polyphenoloxidase, and peroxidase36.
Total antioxidant capacity
Unlike the observed in the tp, that are one of the responsible for the total antioxidant capacity, in this case, differences were observed in the factor analysis that considered moments and treatments for each of the storage temperatures. Untreated peaches conserved at 0 °C for 6 and 27 days had a higher total antioxidant capacity, with values of 0.42 and 0.45 mg aae g-1 fw, respectively, which corresponded to almost twice the values measured in the treated fruit (Figure 3A). Considering the effect of storage time, on untreated fruit preserved at the same temperature (0 °C) an increase was observed at 6 days, a reduction at 18 and finally a new increase at 27 days. On the other hand, in fruit subjected to heat treatment, the total antioxidant capacity remained unchanged during the 27 days of storage. When these fruits were transferred to sl conditions, those treated had a total antioxidant capacity between 20 and 25 % higher than the not treated throughout the entire storage period (Figure 3B). In both treated and untreated fruit, the total antioxidant capacity decreased with time.
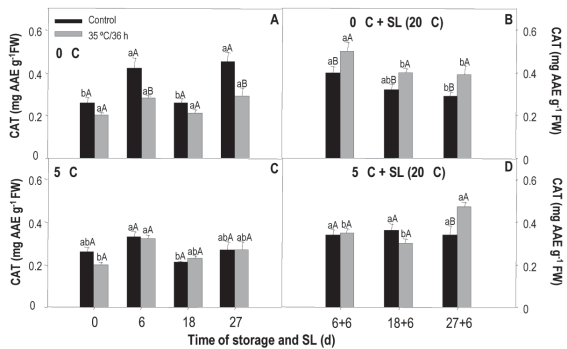
Figure 3: Total antioxidant capacity in nectarines var. Big Top during storage at 0 and 5°C and in shelf life (sl). Values are means (n=3) ± standard error of the mean. Uppercase letters indicate differences for the treatment factor and lowercase for the time factor according to storage temperature (Tukey p<0.05).
When storage was performed at 5 °C, there were no differences between treated and untreated fruit. The average values measured at harvest and end of storage were 0.23 and 0.27 mg aae g-1 fw, respectively (Figure 3C). In this case, the total antioxidant capacity, of both treated and untreated fruit, remained without major variations during refrigerated storage. At the end of the sl period (27+6 days), treated fruit presented antioxidant capacity values 23 % higher than the untreated ones (Figure 2D). Values measured at this time almost doubled those measured during storage.
The main difference between the tp behavior and the total antioxidant capacity was observed in the fruit kept at 0 °C, where in the first case no differences were found and in the second case the untreated fruit presented higher values at 6 and 27 days, indicating that even at this temperature, recommended for the preservation of stone fruit, including nectarines, a stress situation occurs that would stimulate the production of non-phenolic antioxidant compounds. Another aspect that would reinforce this hypothesis is the fact that during sl the treated fruit showed higher levels of total antioxidant capacity at all times of analysis, and not only at 27 days, which was when they differed for its tp content. We can say that, although both compounds participate in the fruit’s antioxidant system, their response to stress is different. Moreover, in a study where the behavior of the TP and the total antioxidant capacity in mango fruits, preserved at a stressful temperature (5 °C for 12 and 24 days) was studied, an increase in the total antioxidant capacity was found compared to those preserved at a non-stressful temperature (13 °C) for the same period, while the tp were not affected37. Vicente and others38 found that the total antioxidant capacity values measured by the dpph method were higher in strawberries treated with hot air (45 °C/3h), which would help the fruit to cope with the overproduction of ROS.
mda Content
Results of the treatment analysis x conservation time indicated that the content of mda increased after 18 days of storage, regardless of temperature and treatment. Values at harvest were between 6 mmol kg-1, while at the end of the storage they reached 25.7 mmol kg-1 fw (Figure 4). For fruit preserved at 0 °C, treated fruits showed a higher content after 6 days of preservation, but at 18 days, the untreated ones presented 1% more mda (Figure 4A). When these fruits were transferred to sl, differences between treatments appeared at 18+6 and 27+6 days (Figure 4B). In the first case, the treated fruit showed higher levels of mda, indicating that their membrane was most affected, and in the second case, the control was the most affected.
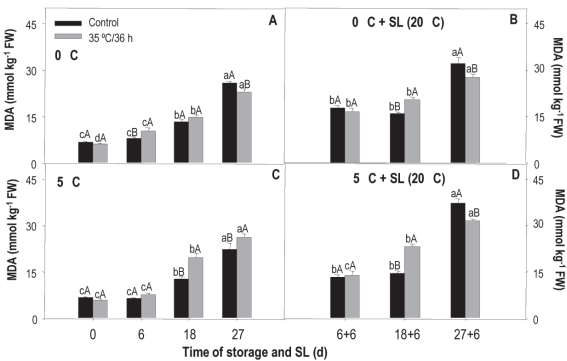
Figure 4: mda content in nectarines var. Big Top during storage at 0 and 5°C and in shelf life (sl). Values are means (n=3) ± standard error of the mean. Uppercase letters indicate differences for the treatment factor and lowercase for the time factor according to storage temperature (Tukey p<0.05).
At 5 °C, differences were observed between treatments after 18 and 27 days of storage and in the 18+6 and 27+6 periods of sl (Figures 4C and 4D). During the storage period, treated fruit presented higher levels of mda at both times, while in sl the behavior was the same as in fruit from 0 °C. Regarding the effect of temperature (temperature x treatment), mda levels measured in treated and untreated fruit were the same at both storage temperatures with values of 17.18 and 16.94 mmol kg-1 fw for 5 and 0 °C, respectively.
mda is a marker of plasma membrane oxidation that accumulates as a result of the increase in ros production and/or the decrease in antioxidant systems39. Huan and others34 found that heat treatments with hot air (38 ºC/3 h) and hot water (48 ºC/10 min) applied to peach fruit (cv. Xiagui 5) determined a lower accumulation of mda during storage, being 15 % less in fruits not treated with heat. The authors associated it with the fact that the treatment was able to suppress the accumulation and overproduction of ros, so there was a lower membrane lipid peroxidation.
In the case of the Big Top variety, and for this study conditions, an erratic behavior was observed that does not allow to conclude on the protective effect of heat treatment on membranes. Although it was expected that the mda content was lower in heat-treated fruit, this was only observed at some times and especially at 0 °C, considered as a less favorable temperature for the occurrence of damage.
Oxidative stress enzyme activity
SOD activity measured in fruit stored at 0 °C shows clear differences between treated and non-treated (treatment x storage time). In the case of the treated, the values fall at 6 and 27 days, while in the control there is an increase at 6 days and from there a very important decrease, of almost 10 times compared to the initial value measured at the remaining evaluation moments (Figure 6A). When comparing treatments, the most noticeable is the considerably greater activity of the treated fruit at 18 and 27 days, evidencing that the heat treatment allowed the enzyme antioxidant protection system to maintain activity and/or even stimulated it. In sl and for both treated and untreated, the activity tended to decrease over time (Figure 5B).
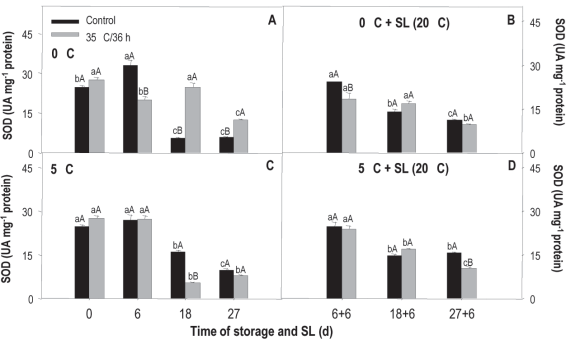
Figure 5: Activity of sod in nectarines var. Big Top during storage at 0 and 5°C and in shelf life (sl). Values are means (n=3) ± standard error of the mean. Uppercase letters indicate differences for the treatment factor and lowercase for the time factor according to storage temperature (Tukey p<0.05).
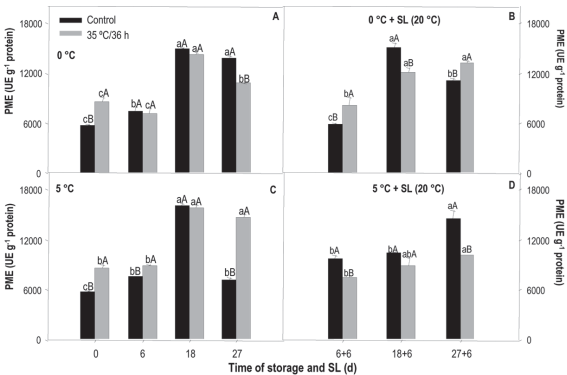
Figure 6: Activity of pme in nectarines var. Big Top during storage at 0 and 5°C and in shelf life (sl). Values are means (n=3) ± standard error of the mean. Uppercase letters indicate differences for the treatment factor and lowercase for the time factor according to storage temperature (Tukey p<0.05).
The behavior observed at 5 °C was different. In this case, the levels fall after 6 days of storage for both treatments, but the activity of sod at 18 and 27 days is lower in fruit that received heat treatment (Figure 5C). In sl, the behavior was the same as that observed in fruit from 0 °C, without major differences between treatments (Figure 5D).
The behavior in sl at both temperatures would be related to the fact that the damage shows to a greater extent in sl, when the temperature rises and metabolic activity is accelerated and, therefore, in these conditions, the enzymatic antioxidant system is exceeded by ros production.
According to the results of the temperature x treatment analysis, at 18 and 27 days of conservation at 0 °C the treated fruit showed an activity 77 % and 37 % higher than those conserved at 5 °C. The exact opposite happened with the untreated, those kept at 5 °C presented a 67 % and 41 % higher activity. Neither of the two conservation conditions showed important differences between treatments during sl (data not shown). This behavior is opposite to the expected since it would be desirable for sod activity to increase in heat-treated fruit.
Jin and others40 found an increase in sod from peaches treated with hot air (38 °C/12 h) but only after 3 weeks of storage at 0 °C. Moreover, Cao and others2 did not observe differences between the activity measured in the untreated and treated up to 21 days of storage at 0 °C, also in peaches treated with hot air (38 °C/12 h). However, after 28 and 35 days of storage, the activity of the sod in the treated fruit exceeded that of the untreated, therefore, an induction period was also necessary for the differences to be observed, as in this study.
The activity of the cat did not show differences between treated and untreated fruit, both at 0 and 5 °C, remained stable throughout the storage, being between 0.3 and 0.4 UA mg-1 of protein (data not shown). In sl, the fruit coming from 0 °C and that had not been treated showed an activity 58 % higher only at the beginning (6+6 days). In the case of those coming from 5 °C and that did not receive heat treatment, the activity was 35 % higher, but only at 27+6 days. When analyzing temperature effect (temperature x treatment), the treated and untreated fruit conserved at 0 and 5 °C did not show differences in storage period or sl (data not shown). In contrast, Khademi and others41 report that in Bright Red persimmon, the activity of the cat in heat-treated fruit (hot water at 45 °C/3 min and 50 °C/20 min) increased during conservation at 1 °C for 30 days. More recently Nasef36 found that cat activity was superior in cucumber fruit treated with hot water for 5 min at both 45 and 55 °C, compared to those that were submerged in water at 25 °C
The activity of the apx measured in fruits stored at 0 °C and untreated was 63 % higher at 18 days with no record of differences between treatments at other times of analysis (data not shown). The same behavior was observed in sl, but only at 27+6 days (data not shown).
For those stored at 5 °C, differences between treatments also appeared at 18 days, although in this case the treated fruit were the ones with the highest activity (data not shown). During the sl, the activity of the apx remained practically unchanged over time, with no differences observed between treatments (data not shown).
The temperature x treatment analysis determined that in the treated fruits the temperature effect showed differences at 18 days, where those conserved at 5 °C showed an activity 71 % higher than those conserved at 0 °C. The untreated fruit only presented differences at the end of the sl period (27+6 days), where the preserved at 0 °C were the ones with the highest activity (data not shown).
apx is part of the ascorbate-glutathione cycle of vegetables and its function is to eliminate H2O2 and regenerate the non-enzymatic antioxidants, ascorbate, and glutathione42. The experiment by Cao and others2 showed a reduction in the activity of the apx with the course of storage, with no differences between peaches treated with heat (38 °C/12 h) and the control up to 21 days of conservation at 0 °C. However, after 28 days the reduction was greater in untreated peaches, so the apx would protect the fruit from free radicals. In tomatoes treated with hot water (52 °C/15 min) and kept at 20 °C for 4 days, no differences were observed with the control, but those kept at 5 °C (stressful temperature) presented a higher activity. In this case, the stress situation triggered the activity of the enzyme42. This inducing effect of the stressful temperature corresponds to that observed in this study, especially in fruit that received heat treatment, where there was greater activity at 5 °C.
Enzyme activity of the wall
The pme activity, obtained from the treatment x storage time analysis, measured in the treated fruit both preserved at 0 °C and 5 °C, almost doubled (14,000 ue g-1) after 18 days of storage, remaining stable at 27 days, except for those stored at 5 °C when a reduction was observed (Figures 6A and 6C).
Control fruit preserved at 0 °C had very similar behavior to that observed in the treated, while at 5 °C the activity increased until 18 days to decrease considerably at the end of the storage, when it reached the initial values (6,000 ue g-1).
Regarding differences between treatments, at 0 °C, the treated showed greater initial activity, but at 27 days the control activity was higher, reaching values of around 13,600 ue g-1. At 5 °C, the heat-treated fruit showed greater activity in almost all the evaluated times, especially at the end of the storage, where the value was almost twice the average in the untreated ones.
In sl, treated fruit from 0 °C showed greater activity at 6+6 and 27+6 days. In those from 5 °C, the control fruit were the most active at all analyzed times (Figures 6B and 6D).
The pg activity measured in the control treatment fruit kept at 0 °C remained unchanged up to 18 days, increasing only at the end of storage (Figure 7A). On the contrary, in the treated fruit a progressive increase was observed up to 18 days, with a reduction observed at 27 days, where the measured values were similar to those measured at the beginning (180 ue g-1). In sl a reduction in activity is observed, always being superior in the control fruit.
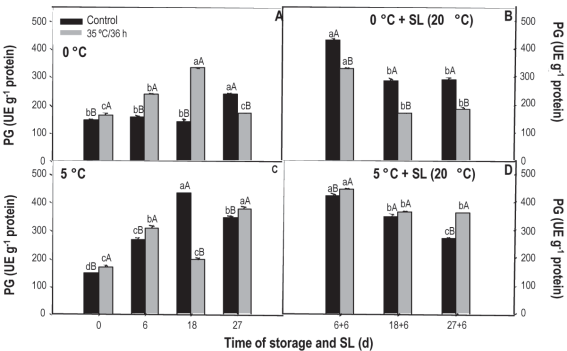
Figure 7: Activity of pg in nectarines var. Big Top during storage at 0 and 5°C and in shelf life (sl). Values are means (n=3) ± standard error of the mean. Uppercase letters indicate differences for the treatment factor and lowercase for the time factor according to storage temperature (Tukey p<0.05).
At 5 °C, the behavior is quite erratic since, at the beginning, 6 and 27 days the activity of the treated fruit is greater, but on day 18 a significant reduction was observed. During sl the activity also decreased over time, but, contrary to what was observed at 0 °C, it was always greater in fruit that received heat treatment.
Although the maturation process slows down during refrigerated preservation, changes continue to occur, so the activity of the pme and pg enzymes is expected to increase with the course of storage. If the activity of the pme increases, but the activity of the pg decreases, galacturonic acid molecules of larger size and reduced methylation accumulate and, as a consequence, can form a gel under esters, leaving dry tissue, without juice or mealy43.
Although in our study we did not evaluate the mealiness degree in fruit, the presence of damage at the different evaluation moments was determined, and the evolution of the enzymes involved in the development of this symptom was evaluated. The results found indicate the development of this disorder, especially in sl and in untreated fruit that, when cut for the other analyzes, showed pasty texture and loss of juiciness. The behavior observed at 5 °C, where the offset was more pronounced in fruits not treated with heat, could indicate a certain palliative effect of heat treatments.
Conclusions
Heat treatment affected the fruit’s antioxidant system, increasing the levels of tp and the total antioxidant capacity in sl. In the enzymatic system, the effect was observed in sod, while in the other enzymes there was practically no treatment effect. The greatest effects were observed when the storage temperature was 0 °C, indicating that without proper temperature management the heat treatment would not work. The high dehydration values indicate that more work should be done on the adjustment of the exposure time, as well as on the confirmation of the results obtained.