Servicios Personalizados
Revista
Articulo
Links relacionados
Compartir
Odontoestomatología
versión impresa ISSN 0797-0374versión On-line ISSN 1688-9339
Odontoestomatología vol.18 no.28 Montevideo nov. 2016
Garchitorena Ferreira, María Inés*
* Assistant Prof. Operative Dentistry 1, Universidad de la República. Uruguay
migarchitorena@gmail.com
Abstract
The focus of the treatment of carious lesions affecting dentin tissue has changed dramatically in recent decades. Knowledge about the etiology of dental caries, dentin pathophysiology and the development of dental biomaterials and adhesive techniques have resulted in minimal intervention treatments.
Given this new alternative for treating dentin lesions, it is necessary to evaluate the potential recovery of dentin through mineralization treatments and the development of bioactive materials that facilitate the repair or regeneration of this tissue by imitating the physiological mechanisms of mineralization and recomposing the tissue’s original mechanical properties. This is done to obtain successful clinical results based on biomimetic treatments.
Keywords: Dentin, Remineralization, Biomimetics.
Received on: 15 Mar 16 – Accepted on: 17 Aug 16
Introduction
Several studies have been conducted to have a better understanding of the biological and microstructural changes in dentin tissue regarding caries lesions and the possibility of remineralizing the tissue involved (1-5).
Dentin is altered on account of acids produced by bacteria, both in its inorganic and organic constituents. These acids lead to dentin tissue demineralization, with the subsequent loss of mineral crystals. They also alter the organic matrix, that is to say, the collagen fibers.
Caries-affected dentin which is preserved in the preparation after eliminating the infected tissue is potentially repairable (6). However, it must be noted that the structure of this residual dentin has specific histologic features on account of the lesion.
A detailed review of the studies conducted to date into dentin remineralization sheds light on several aspects and illustrates the need to continue developing materials with clinical applications that can complement minimal intervention treatment plans.
Development
The modern treatment of carious lesions involves the removal of only the infected external dentin, while internal dentin that can be remineralized is preserved (7).
Therefore, after excavation of the carious lesions, it is likely that the substrate for clinical bonding to restorative materials will be a combination of normal dentin on the periphery and of affected dentin in the center of the lesion. The mineral distribution of the caries-affected dentin is highly variable, and the depth of the lesion can extend hundreds of microns below the excavated surface.
The biomimetics treatment of caries-affected tissues involves guiding remineralization, following or imitating the physiological mechanisms of tissue mineralization. Therefore, knowing how these processes naturally occur in odontogenesis and understanding cellular signaling by bio-active components released from the dentin matrix during injury may help develop techniques and materials to facilitate the biomechanical recovery of the dentin affected by pathological processes, surgical procedures and restorative materials (8).
Caries-affected dentin regeneration is a desirable clinical approach which is opposed to traditional practice whereby the tissue is fully removed from the dental preparation. The partial removal of carious dentin, when health condition and pulp response are correctly diagnosed, allows for the necessary biological conditions to prevent the lesion from progressing and to promote pulp defense mechanisms. The hermetic seal of the restoration is essential for a conservative treatment to be successful, within comprehensive planning based on minimal intervention principles (9-12).
However, dentin tissue recovery is a complex process as it involves the reconstitution of two different phases: type-I organic collagen and inorganic apatite, which have a specific spatial connection. This composite structure of dentin is denatured on account of demineralization and the subsequent breakdown of collagen polymerization when facing the acid conditions of carious lesions. This means that remineralization on its own is insufficient for the full recovery of demineralized carious dentin. It is also necessary to regenerate the structure of the collagen matrix, and both stages must be connected in a specific way. The recovery of denatured collagen can be crucial to re-establish the micromechanical properties of carious dentin through intrafibrillar remineralization (13).
Some studies show that the deeper sites need to contain residual minerals for the dentin affected by carious lesions to be remineralized. This should be intrafibrillar mineral to allow for nucleation and growth during remineralization (1, 4). It has been determined that remineralization occurs through the growth of crystals that tend to increase in size and eventually bond, thus forming tissue that is more homogeneously remineralized (1).
Remineralized tissue that has restored mechanical properties under physiological conditions indicates that mineral crystallites are closely linked or even chemically bound to the collagen matrix. The quality of the dentin will depend on the tissue’s characteristics: microstructure, mineral density and location of minerals in the organic matrix (1, 14).
Biomaterials are elements used to repair or replace damaged organs or tissues. Research on biomaterials began in the 1960s, as the discipline started to develop as a science. At first they were inert materials, and as of the 1980s a second generation began: bioactive materials. These materials can replace tissues.
Nowadays scientific research develops cells and tissues, that is to say, regenerating biomaterials. These promote cell activation and aid the regeneration of specific tissues. This is how biomaterials science intersects with tissue engineering, an area of bio-engineering that focuses on the recovery of biological functions, as these materials require solid or gel-based supports or scaffolds to guide cell proliferation. To do this, supports must be porous and biodegradable. Polymer or ceramic supports, or polymer-ceramic supports are used, and research currently focuses on developing nanoscale supports. Their ceramic phase is formed by bioactive glass, containing silicate (SiO2), calcium oxide (CaO) and phosphorus oxide (P2O5).
In recent years, biomimetic approaches have been adopted to develop nanomaterials to be included in oral health products. For instance, these can be mouthwashes and toothpastes containing nano-apatites that interact with biofilm and precipitate on the tooth surface. They can also be products containing nanomaterials to remineralize early enamel sub-micrometric lesions. However, research on the use of nanomaterials in larger cavities is still under way (15).
Conventional remineralization of the carious dentin often involves using solutions with calcium and phosphate ions in various fluoride concentrations. It has been well established that conventional remineralization does not occur through spontaneous nucleation of the mineral in the organic matrix, but rather through the growth of residual apatite crystals in partially demineralized dentin. The conventional remineralization strategy relies on epitaxial growth on existing apatite crystals. If there are none or very few residual crystals, there is no remineralization. The mineral content of the surface layer of the lesion impacts the characteristics of the resulting remineralization, including its location and mineral deposition density (2).
In turn, guided tissue remineralization resorts to biomimetic analogs of dentin matrix phosphoproteins to induce amorphous calcium phosphate (ACP) nanoprecursors in the internal compartments of collagen fibrils (16). This strategy is independent from apatite crystallites that may have remained. This biomimetic remineralization process is a bottom-up approach used to create nanocrystals that are small enough to fit in the gaps between adjacent collagen molecules and establish a hierarchical order regarding mineralized collagen (Fig. 1). Both totally and partially demineralized dentin, with reconstituted type-I collagen fibrils that are completely devoid of matrix phosphoproteins have been successfully remineralized with this strategy (2).
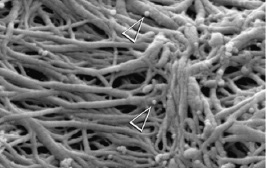
Fig. 1 ACP nanoprecursors are indicated with arrows (Tay 2008)
Some people are working on the experimental stages, and, in some cases, selling various dental materials used to recompose dentin tissue.
Bioactive glasses are surface-active materials which minerals can bind to. The basic components of bioactive glasses are calcium oxide, sodium, phosphorus and silica (17).
Although most bioactive glass information has been acquired through bone research, this material has also been used in dentistry, and there is growing interest in this area, especially for dentin mineralization or remineralization (18).
Bioactive glasses are thought to form a bioactive layer at the interface in contact with live tissue called hydroxyapatite (HCA), which is equivalent to the mineral phase of hard human tissues (19).
A significantly higher remineralization rate has been observed with the use of bioactive glass nanoparticles as opposed to micrometric glass particles. This confirms the importance of particle size in the clinical applications of bioglasses (Fig. 2).
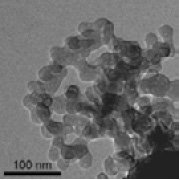
Fig. 2 TEM image of a bioactive glass nanoparticle (Vollenweider 2007)
Polyelectrolytes are polymers whose repeating units bear an electrolyte group. These groups dissociate in aqueous solutions, making the polymers charged. Charged molecular chains, commonly present in several soft matter systems, play a key role in determining the structure, stability and interactions of various molecular assemblies. Both natural and synthetic polyelectrolytes are used in a variety of industries. Polyvinylphosphonic acid (PVPA) is a polyelectrolyte that has been used as a biomimetic analog for phosphoproteins like Dentin matrix acidic phosphoprotein 1 (DMP1) and dentin phosphoprotein (DPP) (20).
Amorphous calcium phosphate (ACP) is an HA precursor, this being the final and stable product in the precipitation of calcium and phosphate ions in neutral or alkaline solutions. Regarding the large scope of solution conditions in which precipitation occurs spontaneously, amorphous calcium phosphate precedes the occurrence of HA. This is why they have been studied as a filler phase in polymeric materials, stimulated by its excellent biocompatibility and the release of calcium and phosphate ions (21).
However, in their application for dental materials, the relatively high solubility of ACP and its quick conversion to ACP in an aqueous environment might entail limitations regarding structural, mechanical and chemical stability (21). The nanocomplex casein amorphous calcium phosphate (RecaldentTM (CPP-ACP) is technology based on ACP stabilized by casein phosphopeptides (CPP), making them a metastable complex. This technology has shown anticariogenic activity in both in situ studies and clinical trials. Additionally, a significant increase has been observed in the levels of calcium and phosphate ions on supragingival plaque when mouthwash is used. Also, the in situ remineralization of enamel subsuperficial lesions is promoted (22). These properties might make ACP an effective mineralizing agent (21). ACP bioactivity may prove particularly useful when included in composite resins, sealants and adhesives in order to prevent tooth demineralization and to actively promote remineralization. According to a study published in 2003, the inclusion of silica (Si) and/or zirconia (Zr) in the ACP improves the duration of the release of mineral ions through the slower conversion to HAP observed in the composite (23).
Calcium silicate cements, called mineral trioxide aggregate cements (MTA, such as ProRoot MTA, MTA Angelus, Tech Biosealer) are Portland cement derivatives. They are used as dentistry materials for different clinical applications, particularly in endodontics. Calcium silicate cements are hydrophilic materials that can tolerate humidity and that harden with biofluids (blood, plasma, saliva, dentinal fluid). These materials can release calcium and hydroxyl ions (alkalizing activity) into surrounding fluids, thus creating the conditions necessary for apatite formation (24).
An in vitro study published in 2011 showed the bioactivity of experimental composite resins containing calcium-silicate powder (24). Selecting the correct hydrophilic resin to prepare experimental compounds was essential to provide the water absorbing capacity and bioactivity properties: the absorption of small amounts of water leads to the hydration of calcium-silicate filling materials, which allows for calcium release and apatite formation. Additionally, the hydroxyl ions that are released during hydration can create unfavorable conditions for bacteria survival and proliferation. Antibacterial properties are necessary mainly in the dentin-restoration interfacial area.
Although glass-ionomer cements contain calcium and phosphate, they show no bioactivity. Besides, there is a wealth of information on the positive effects of fluoride on the enamel, but there is no data proving the efficacy of fluoride ions to induce mineralization in demineralized dentin or to contribute to the nucleation of new apatite crystallites in dentin with no residual apatite, with the use of classic glass ionomers (24).
It would be beneficial if glass-ionomer cement had bioactivity, as nowadays biomaterials with therapeutic or bioactive functions tend to be developed, which add to its inherent properties (18).
Recently a technique that involves the guided formation of a layer of fluorapatite that resembles enamel has been developed. This is done on a mineral substrate and has the potential to enhance the remineralization of superficial enamel and/or exposed dentin defects. This technique, Biomimetic Mineralization (BIMIN), uses calcium ions diffusion from a solution in a glycerin-enriched gel with phosphate and fluoride ions. When the conditioned gel is in direct contact with the tooth’s exposed surface, a mineral layer firmly adhered to the tooth’s surface is formed within eight hours. The recent application of BIMIN in a clinical feasibility study showed the formation of a fluorapatite deposit on tooth enamel. Biomimetic mineralization is based on the principle of ion crystallization from oversaturated solutions and involves the controlled diffusion of calcium, phosphate and fluoride ions onto the tooth’s surface. It has been proven that this technique generates fluorapatite on enamel prisms, which was confirmed by X-ray diffraction. More recently, the in vivo biomimetic mineralization effect on human enamel has been reported. In this case, night application led to the formation of a layer that resembled enamel on the tooth’s surface (25). A possible BIMIN application is the precipitation of minerals on dentin surfaces, thus obstructing open tubules. This makes it possible for this method to have a clinical application for treating sensitivity, erosion and other conditions (25).
Discussion
Several studies have reported, in the last few decades, scientists’ efforts to remineralize dentin, including the use of phosphoproteins (26), casein amorphous calcium phosphate phosphopeptides (21, 27), bioactive glass particles (28), colloidal nano-beta-tricalcium phosphate (13) and polyelectrolytes containing carboxylic acids (20). These studies have reported relative success in the mineral formation where the dentin lesion is located (1).
The ideal biomaterial should promote reactive dentin deposition and also remineralize the affected dentin that remains after the partial removal of the carious lesions.
Mineral formation is a necessary, but may not be a sufficient, condition for reestablishing dentin functionality after remineralization treatments (14). Mineral concentration in itself is insufficient to evaluate the success of current remineralization strategies. Mechanical recovery seems to be partially reestablished when the mineral is incorporated into the structure, but its connection with the matrix is different from physiological recovery (1). Re-establishment of functionality requires both the formation of extrafibrillar and intrafibrillar minerals in the gap regions of the collagen fibrils (14). Therefore, intrafibrillar remineralization in particular is essential to restore the mechanical properties of dentin (2, 3, 14, 29).
It is still difficult for new advances in remineralizing biomaterials to be translated into materials that are available on the market. However, research is promising and predicts a not so distant future where clinicians can have a large range of bioactive materials at their disposal to help them in their conservative treatments in restorative dentistry (Table 1).
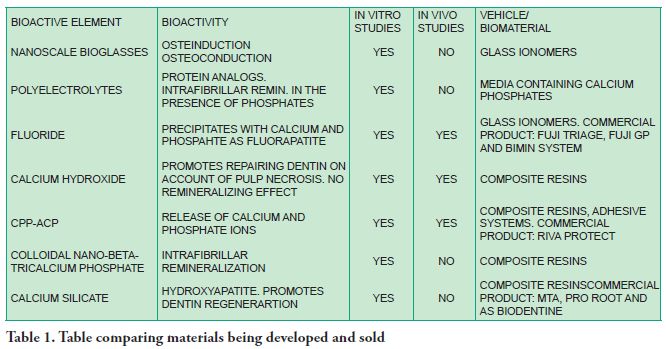
Final remarks
There is currently no clinical product available on the market to achieve biomimetic remineralization (25). However, great progress is being made into biomaterials, especially by applying nanotechnology and tissue engineering.
Nanotechnology has made it possible to develop materials with bioactive properties that can act at nanoscale levels, activating cells and tissues to promote tissue regeneration (30).
The development of dentin regenerating biomaterials will make it possible to recompose the complex structure of this tissue, and it will also be able to recover when facing the masticatory effort. This will contribute to improving restorative dentistry, regarding both the maximum conservation of healthy tissue and tissue regeneration.
Referencias
2. Dai L, Liu Y, Salameh Z, Khan S, Mao J, Pashley DH, Tay FR. Can caries- affected dentin be completely remineralized by guided tissue remineralization? Dent Hypotheses. Jan 2011; 2 (2): 74-82
3. Kinney JH, Habelitz S, Marshall SJ, Marshall GW. The importance of intrafibrillar mineralization of collagen on the mechanical properties of dentin. J Dent Res. 2003; 82 (12) 957-61
4. Ten Cate JM. Remineralization of caries lesions extending into dentin. J Dent Res. 2001; 80 (5): 1407-11.
5. Veis A. Biomineralization: on the trail of the phosphate. Part I: Chance encounters and the path to dentin. J Dent Res. 2003; 82 (12): 941-43.
6. Bresciani E, Wagner WC, Navarro MFL, Dickens SH, Peters MC. In vivo dentin microhardness beneath a calcium phosphate cement. J Dent Res. 2010 89(8) 836- 41.
7. Mehdawi I, Abou Neel EA, Valappil SP, Palmer G, Salih V, Pratten J, Spratt DA, Young AM. Development of remineralizing, antibacterial dental materials. Acta Biomater. 2009; 5: 2525-39.
8. Smith AJ, Tobias RS, Murray PE. Transdentinal stimulation of reactionary dentinogenesis in ferrets by dentine matrix components. J Dent. 2001; 29: 341-46.
9. Bjorndal L, Reit C, Bruun G, Markvart M, Kjaldgaard M, Nasman P et al. Treatment of deep caries lesions in adults: randomized clinical trials comparing stepwise vs. direct complete excavation, and direct pulp capping vs. partial pulpotomy. Eur J Oral Sci. 2010; 118: 290-7.
10. Alves L, Fontanella V, Damo A, Ferreira de Oliveira E, Maltz M. Qualitative and quantitative radiographic assessment of sealed carious dentin: a 10-year prospective study. Oral Surg Oral Med Oral Pathol Oral Radiol Endod. 2010 Jan;109(1):135-41.
11. Schwendicke F. Incomplete Caries Removal : A Systematic Review and Meta-analysis. J Dent Res 2013; 92 (4): 306-14.
12. Maltz M, Oliveira EF, Fontanella V, Carminatti G. Deep caries Lesions after incomplete dentine caries removal: 40-month follow-up study. Caries Res 2007; 41(6):493-6.
13. Shibata Y, He LH, Kataoka Y, Miyazaki T, Swain MV. Micromechanical property recovery of human carious dentin achieved with colloidal nano-beta-tricalcium phosphate. J Dent Res. 2008; 87:233–7
14. Bertassoni LE, Habelitz S, Kinney JH, Marshall SJ, Marshall GW. Biomechanical perspective on the remineralization of dentin. Caries Res. 2009; 43: 70-7.
15. Hannig M, Hannig C. Nanomaterials in preventive dentistry. Nat Nanotechnol 2010; 5:565–9.
16. Gu L, Kim YK, Liu Y, Ryou H, Wimmer CE, Dai L, Arola DD, Looney SW, Pachley DH, Tay FR. Biomimetic analogs for collagen biomineralization. J Dent Res.2011; 90 (1): 82-7.
17. Yli-Urpo H, Narhi M, Narhi T. Compuond changes and tooth mineralization effects of a glass ionomer cements containing bioactive glass (S53P4), an in vivo study. Biomater. 2005; 26: 5934-41.
18. Prabhakar AR, Paul J, Besappa N. Comparative evaluation of the remineralizing effects and surface microhardness of glass ionomer cements containing bioactive glass (S53P4): an in vitro study. Int J Clin Ped Dent. 2010; 3 (2): 69-77.
19. Salonen JI, Arjasamaa M, Tuominen U, Behbehani MJ, Zaatar EI. Bioactive glass in dentistry. J Minim Interv Dent. 2009; 2(4): 208-18.
20. Tay FR, Pashley DH. Guided tissue remineralization of partially demineralized human dentin. Biomater. 2008; 29: 1127-37.
21. Skrtic D, Antonucci JM, Eanes ED. Amorphous calcium phosphate-based bioactive polymeric composites for mineralized tissue regeneration. J Res Natl Inst Stand Technol . 2003; 108: 167-82.
22. Reynolds EC. Calcium phosphate-based remineralization systems: Scientific evidence? Austr Dent J 2008; 53:268-73.
23. Dickens SH, Flaim GM, Takagi S. Mechanical properties and biochemical activity of remineralizing resin-based Ca-PO4 cements. Dent Mater.2003;19:558-66.
24. Gandolfi MG, Taddei P, Siboni F, Modena E, De Stefano ED, Prati C. Biomimetic remineralization of human dentin using promising innovative calcium silicate hybrid “smart”materials. Dent Mater. 2011. 27. 1055 69.
25. Guentsch A, Seidler K, Nietzsche S, Hefti AF, Preshaw PM, Watts DC, Jandt KD, Sigusch BW. Biomimetc mineralization: long-term observations in patients with dentin sensitivity. Dent Mater. 2012; 28: 457-64.
26. Saito T, Yamauchi M, Crenshaw MA. Apatite induction by insoluble dentin collagen. J Bone Miner Res. 1998; 13(2): 265-70.
27. Rahiotis C, Vougiouklakis G. Effect of a CPP-ACP agent on the demineralization and remineralization of dentine in vitro. J Dent. 2007; 35: 695-98.
28. Vollenweider M, Brunner TJ, Knecht S, Grass RN, Zehnder M, Imfeld T, Stark WJ. Remineralization of human dentin using ultrafine bioactive glass particles. Acta Biomater. 2007; 3: 936-43.
29. Kim YK, Yiu CKY, Kim JR, Gu L, Kim SK, Weller RN, Pashley DH, Tay FR. Failure of a glass ionomer to remineralize apatite depleted dentin. J Dent Res. 2010. 89(3) 230 35.